Thank you for visiting nature.com. You are using a browser version with limited support for CSS. To obtain the best experience, we recommend you use a more up to date browser (or turn off compatibility mode in Internet Explorer). In the meantime, to ensure continued support, we are displaying the site without styles and JavaScript.
Scientific Reports volume 14, Article number: 8024 (2024 ) Cite this article 5g Network Components
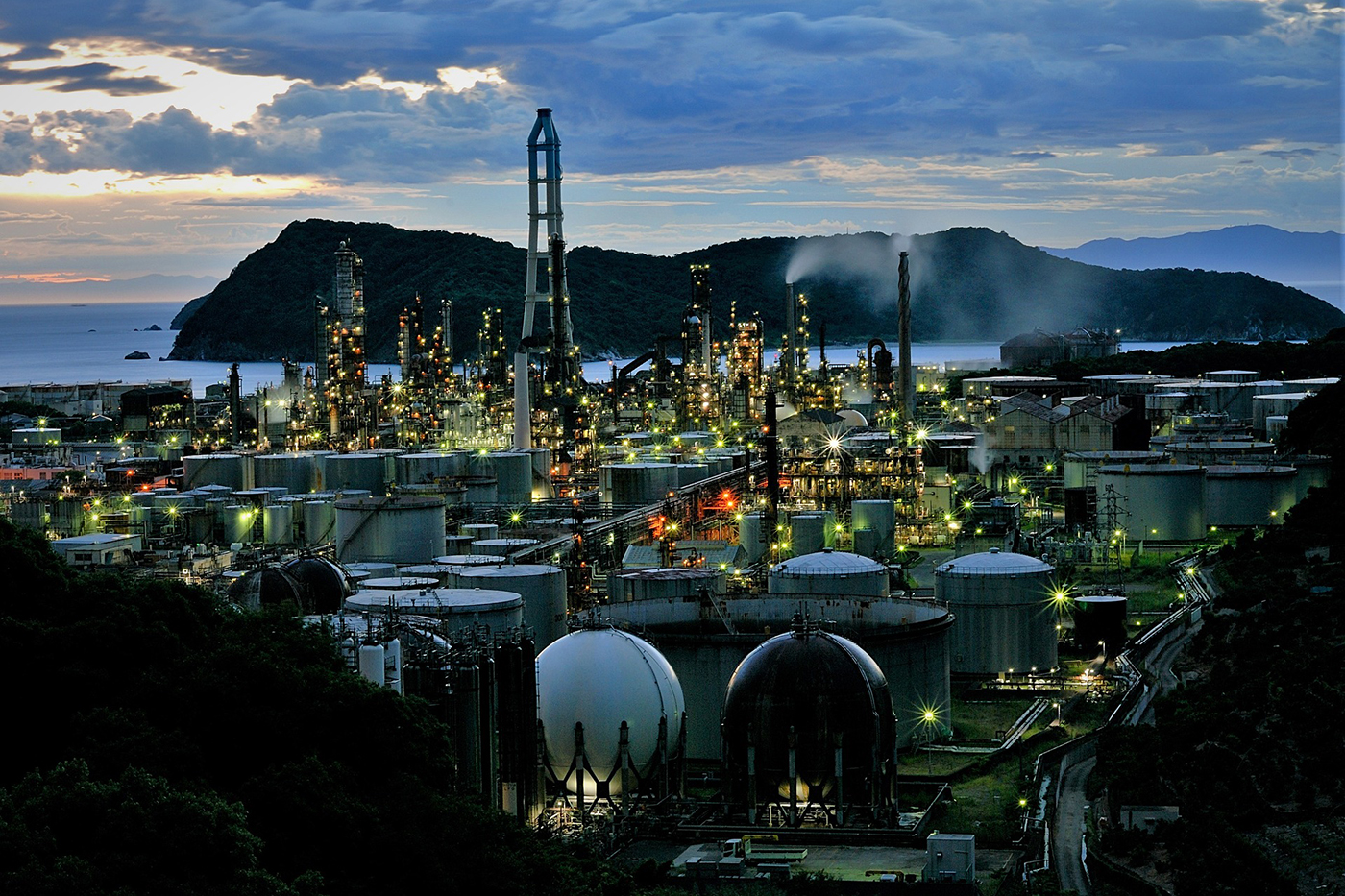
Diabetes mellitus is recognized as a major predisposing factor for Moraxella keratitis. However, how diabetes mellitus contributes to Moraxella keratitis remains unclear. In this study, we examined Moraxella keratitis; based on the findings, we investigated the impact of advanced glycation end products (AGEs) deposition in the cornea of individuals with diabetic mellitus on the adhesion of Moraxella isolates to the cornea. A retrospective analysis of 27 culture-proven cases of Moraxella keratitis at Ehime University Hospital (March 2006 to February 2022) was performed. Moraxella isolates were identified using matrix-assisted laser desorption ionization time-of-flight mass spectrometry. Among the patients, 30.4% had diabetes mellitus and 22.2% had the predominant ocular condition of using steroid eye drops. The species identified were Moraxella nonliquefaciens in 59.3% and Moraxella lacunata in 40.7% of patients. To investigate the underlying mechanisms, we assessed the effects of M. nonliquefaciens adherence to simian virus 40-immortalized human corneal epithelial cells (HCECs) with or without AGEs. The results demonstrated the number of M. nonliquefaciens adhering to HCECs was significantly increased by adding AGEs compared with that in controls (p < 0.01). Furthermore, in the corneas of streptozotocin-induced diabetic C57BL/6 mice treated with or without pyridoxamine, an AGE inhibitor, the number of M. nonliquefaciens adhering to the corneas of diabetic mice was significantly reduced by pyridoxamine treatment (p < 0.05). In conclusion, the development of Moraxella keratitis may be significantly influenced by the deposition of AGEs on the corneal epithelium of patients with diabetes mellitus.
Moraxella species were isolated from a patient with conjunctivitis by Morax in 1896, and the following year, Axenfeld isolated them alone1 They present as Gram-negative, twin-short bacilli and are common in the mucous membranes of the human oral cavity, nasal cavity, and pharynx2,3 Moraxella species cause otitis media, sinusitis, laryngitis, pneumonia, and meningitis, and Moraxella catarrhalis is the primary causative agent of respiratory tract infections2,4,5,6,7 In the field of ophthalmology, Moraxella species are etiological agents of various infectious diseases, such as conjunctivitis, keratitis, blepharitis, and endophthalmitis, and various Moraxella species, including M. catarrhalis, have been reported as causative organisms8,9,10,11,12,13,14,15,16,17,18,19,20.
Moraxella species are the second most significant Gram-negative rods of infectious keratitis, following Pseudomonas aeruginosa21,22,23 Diabetes mellitus has been previously reported as a major systemic predisposing factor for Moraxella keratitis9,11,12,14,15,16,18,19,20 Diabetes mellitus affects the tear fluid, such as decreased volume, increased glucose concentration, intracorneal nerve abnormalities, and metabolic abnormalities in the corneal epithelium, resulting in diabetic keratopathy24 Metabolic abnormalities cause an increase in the concentrations of aldose reductase and deposition of advanced glycation end products (AGEs) in diabetic keratopathy25,26 The Maillard process, which is the nonenzymatic glycation of proteins, produces AGEs as byproducts27 AGEs accumulate throughout the body and cause various diseases, including cardiovascular and renal diseases28 The accumulation process of AGEs is caused by cell metabolic dysfunction and changes in various macromolecule structures, which impact the physiological activities of various eye components29,30,31,32,33 Furthermore, the formation of AGE is closely related to aging eye pathologies, such as cataracts and diabetic retinopathy29,30,31,32,33 AGE deposition in corneal layers, including epithelium, Bowman's layer, and endothelium, has been shown in monkeys, mice, and humans with diabetes mellitus29,34,35 According to Kaji et al.29, AGE deposition on laminin in the corneal epithelial basement membrane results in impaired laminin extension and corneal epithelial defects. Shi et al.36 found that AGEs induce apoptosis of corneal epithelial cells. Therefore, AGEs have various effects on the cornea and are associated with infection. Ozer et al.37 reported that AGEs play a role in bacterial adhesion to the bladder epithelium in urinary tract infections. To the best of our knowledge, a link between AGEs and ocular infections has never been reported. Bacterial adhesion to biological surfaces is essential for the development of infectious diseases38,39. We hypothesize that increased adhesion of Moraxella to the corneal epithelium in patients with diabetes mellitus may be a reason why diabetes mellitus is a major systemic predisposing factor for Moraxella keratitis. In this study, we retrospectively reviewed the species and predisposing factors for Moraxella keratitis at our institution and focused on AGE deposition on the cornea of patients with diabetes mellitus. We examined changes in the ability of Moraxella nonliquefaciens, which is considered a major species associated with Moraxella keratitis, to adhere to the corneal epithelium in the presence or absence of AGEs.
Among the 27 patients included in this study, whose ages ranged from 28 to 94 years, 16 were male and 11 were female. Using matrix-assisted laser desorption ionization time-of-flight mass spectrometry (MALDI-TOF MS), we successfully identified each isolate from the specimens of Moraxella keratitis. This led to a distinction between two prevalent Moraxella species: M. nonliquefaciens in 59.3% (16 isolates) and Moraxella lacunata in 40.7% (11 isolates) of cases. The majority of patients (77.8%) significantly presented with ocular-predisposing factors, as detailed in Table 1. Notably, 22.2% of these patients used steroid eye drops, and common ocular conditions like bullous keratopathy, dry eye, penetrating keratoplasty, lagophthalmos, and trauma were observed in 7.4% of the cases. Additionally, our investigation of systemic predisposing factors in 23 out of the 27 patients revealed that 60.9% of them had relevant systemic risk factors that included diabetes mellitus in 30.4%, use of anticancer drugs in 26.1%, and Sjögren’s syndrome in 4.3% of the cases.
The effect of AGEs on the adhesion of M. nonliquefaciens to human corneal epithelial cells (HCECs) immortalized by SV 40 was examined. The number of adherent M. nonliquefaciens was compared between HCECs supplemented with 100 µg/mL advanced glycation end product–bovine serum albumin (AGE-BSA) and those supplemented with bovine serum albumin (BSA) alone. The number of adherent M. nonliquefaciens was significantly higher in HCECs supplemented with 100 µg/mL AGE-BSA (p < 0.05 for the two-tailed unpaired t-test; Fig. 1).
Effects of AGEs on the ability of Moraxella nonliquefaciens to adhere to HCECs. The number of adherent M. nonliquefaciens was significantly higher in HCECs with AGE-BSA. Two-tailed unpaired t-test, *p < 0.01. The mean ± SEM of CFU/mL for each group is shown by the central horizontal lines bisected by error bars (n = 10 wells/group). AGE, advanced glycation end product; BSA, bovine serum albumin; HCEC, human corneal epithelial cells; SEM, standard error of the mean.
The immunohistochemistry (IHC) results showed AGE immunoreactivity in the corneas of diabetic mice that consumed pyridoxamine, diabetic mice, age-matched wild-type mice, and young wild-type mice (Fig. 2). In diabetic mice, AGE reactivity was diffusely observed in all layers of the corneal epithelium and endothelial cells. In contrast, in diabetic mice that consumed pyridoxamine, marginal AGE reactivity was observed in the basal layer of the corneal epithelium and endothelial cells. In age-matched wild-type mice, AGE reactivity was observed in the deep corneal epithelium, basal layer of the corneal epithelium, and endothelial cells. Young wild-type mice showed no AGE reactivity.
AGE immunoreactivity in the corneas of diabetic mice that consumed PM, diabetic mice, age-matched wild-type mice, and young wild-type mice. Diabetic mice treated with PM showed almost no AGE reactivity in the cornea. Diabetic mice showed AGE reactivity diffusely in all layers of the corneal epithelium and endothelial cells. Age-matched wild-type mice showed moderate AGE reactivity in the deep corneal epithelium, basal layer of the corneal epithelium, and endothelial cells. Young wild-type mice showed no AGE reactivity. AGE, advanced glycation end product; DM, diabetes mellitus; Endo, endothelium; Epi, epithelium; PM, pyridoxamine; St, stroma; WT, age-matched wild-type; YWT, young wild-type.
The number of adherent M. nonliquefaciens was significantly higher in the corneas of diabetic mice than in those of diabetic mice that consumed pyridoxamine (p < 0.05 for two-tailed unpaired t-test; Fig. 3). In contrast, no significant difference in the number of adherent M. nonliquefaciens was observed between the corneas of diabetic mice and those of age-matched wild-type mice, although the number of adhesions tended to be higher in diabetic mice. The number of adherent M. nonliquefaciens was significantly higher in the corneas of age-matched wild-type mice than in those of young wild-type mice (p < 0.05, two-tailed unpaired t-test; Fig. 4).
The effect of AGEs on the ability of Moraxella nonliquefaciens to adhere to the mouse corneas. The number of adherent M. nonliquefaciens was significantly higher in the corneas of diabetic mice than those with diabetes mellitus treated with PM. Two-tailed unpaired t-test, *p < 0.05. There was no significant difference in the number of adhesions between diabetic mice and age-matched wild-type mice. Two-tailed unpaired t-test, p = 0.09. The mean ± SEM of CFU/mL for each group is shown by the central horizontal lines bisected by the error bars (n = 5 mice/group). AGE, advanced glycation end product; DM, diabetes mellitus; PM, pyridoxamine; WT, age-matched wild-type; SEM, standard error of the mean.
The effect of AGEs on the ability of Moraxella nonliquefaciens to adhere to the mouse corneas. The number of adherent M. nonliquefaciens was significantly higher in the corneas of age-matched wild-type mice than in those of young wild-type mice. Two-tailed unpaired t-test, *p < 0.05. The mean ± SEM of CFU/mL for each group is shown by the central horizontal lines bisected by the error bars (n = 5 mice/group). AGE, advanced glycation end product; WT, age-matched wild-type; YWT, young wild-type; SEM, standard error of the mean.
Clinical features of Moraxella keratitis were reviewed retrospectively, demonstrating M. nonliquefaciens as the main etiological agent of corneal infection associated with diabetes mellitus. The identification of Moraxella species is difficult in laboratories because of their similar biochemical properties12. Recently, a quick and accurate approach for identifying microbes has emerged: MALDI-TOF-MS40,41. Thus, identifying the species of Moraxella responsible for keratitis is now possible. In this study, only two species, M. nonliquefaciens and M. lacunata, were identified in keratitis. These species have been reported to be major causative species of Moraxella keratitis in previous reports12,16,17,18,19. In support of our results, LaCroce et al. reported that M. nonliquefaciens was the most frequently identified organism in cases of Moraxella keratitis (80.5%)17. Thus, M. nonliquefaciens is considered a major species that causes Moraxella keratitis. Moreover, these two species do not reside on the ocular surface; rather, they are endemic in the oral cavity and upper respiratory tract and can be transient bacteria on the ocular surface42.
Diabetes mellitus was previously reported as a major predisposing factor for Moraxella keratitis, with frequency ranging from 7.3% to 23.3%9,11,12,14,15,16,18,19,20. Similar to other studies, 30.4% of the patients in this study had diabetes mellitus.
Adhesion is essential for bacteria to develop an infection, and the same is true for infectious keratitis38,39. Reichert et al. reported that the difference in pathogenicity of bacteria in infectious keratitis is related to their ability to adhere to the corneal epithelium43. To the best of our knowledge, the ability of Moraxella to adhere to the corneal epithelium has not yet been evaluated. Pseudomonas aeruginosa, a Gram-negative rod bacterium, has type 4 pili required for adhesion to corneal epithelial cells44. Moraxella species also have type 4 pili45,46. Ozer et al. reported that the binding of AGEs to the pili of uropathogenic Escherichia coli (UPEC) increased the adhesion of UPEC to the urothelium37. We hypothesized that similar changes might be occurring in the cornea. The adhesion of Moraxella to the corneal epithelium may be increased by binding the pili of Moraxella to AGEs in the corneal epithelium deposited by diabetes mellitus. To clarify this hypothesis, we examined the adhesion of M. nonliquefaciens to the corneal epithelium in vitro using HCECs and ex vivo using the corneas of mice.
The number of adherent M. nonliquefaciens to HCECs was significantly increased by the addition of AGEs to HCECs (Fig. 1). Ozer et al. reported that in vitro binding of type 1 pili to several AGEs enhances the adhesion of UPEC to the urothelium, and Fim H, an adhesin, may be involved in type 1 pili binding to AGEs37. Type 1 pili are classified as chaperone-usher pili, and Gram-negative rods universally express chaperone-usher pili on their outer surfaces47,48. Pili play an essential role in the adhesion of bacteria to host tissues, particularly the adhesin at the tip of the pilis49. Thus, we hypothesized that the adhesion of M. nonliquefaciens to the corneal epithelium is facilitated by binding of these pilis to AGEs deposited in the corneal epithelium. Further studies are required to determine whether AGEs bind to the pili of M. nonliquefaciens.
To confirm the possibility that AGEs enhance the ability of M. nonliquefaciens to adhere to the corneal epithelium, we induced diabetes mellitus in mice in which AGEs were deposited in the cornea and conducted ex vivo experiments using the corneas of these mice. In this study, IHC results showed that AGEs were highly deposited in the entire corneal epithelium, epithelial basement membrane, and endothelial cells in diabetic mice (Fig. 2). This is consistent with previous studies that used mice and monkeys with diabetes mellitus34,35. The high blood glucose level in diabetic mice is believed to cause a Maillard reaction due to excess sugar, resulting in the deposition of high levels of AGEs50. AGEs have also been reported to accumulate with aging51,52. Our results support these previous reports, as moderate AGE deposition was observed in the cornea of age-matched wild-type mice. In this study, pyridoxamine, which is a natural type of vitamin B, was used as an AGE inhibitor. Pyridoxamine inhibits the formation of AGEs from Amadori intermediates by blocking the Maillard reaction53. Pyridoxamine inhibits the deposition of AGEs in various animal studies35,37,54 and mouse cornea35. In this study, we confirmed that pyridoxamine suppressed the deposition of AGEs in the cornea of mice with streptozotocin (STZ) -induced diabetes mellitus (Fig. 2). Ex vivo examination demonstrated that M. nonliquefaciens adhered more readily to the corneal epithelium in the following order: diabetic mice, age-matched wild-type mice, and diabetic mice that consumed pyridoxamine. Furthermore, the number of bacteria adhering to the cornea was significantly higher in diabetic mice than those that consumed pyridoxamine (Fig. 3). These results are consistent with the degree of AGE deposition in each mouse cornea, as demonstrated by IHC examination, confirming that the number of adherent M. nonliquefaciens in the cornea increases with AGE deposition. This suggests that AGE deposition in the cornea affects the ability of M. nonliquefaciens to adhere to the corneal epithelium. In this study, diabetic mice and age-matched wild-type mice showed no significant difference in the number of Moraxella adhesions to the cornea, although that number tended to be higher in diabetic mice. The mice in this study were 24 weeks old, which may affect AGE deposition in the cornea and bacterial adhesion. IHC of corneas removed from young wild-type mice (8-week-old) showed no AGE immunoreactivity (Fig. 2). This suggests that aging also induces the accumulation of AGEs in the cornea. An ex vivo examination of age-matched and young wild-type mice showed a significantly higher number of M. nonliquefaciens adhesions in the corneas of age-matched wild-type mice (Fig. 4). This finding is consistence with the results of IHC. Moraxella keratitis occurs more frequently in the elderly11,22, possibly related to AGE deposition in the cornea due to aging.
Some limitations of this study should be acknowledged. First, how M. nonliquefaciens adheres to AGEs in the corneal epithelium remains uncertain. Second, we have not investigated whether changes in the cornea with diabetes mellitus other than AGE deposition affect the ability of M. nonliquefaciens to adhere to the corneal epithelium. Further studies are necessary to clarify the relationship between M. nonliquefaciens and diabetes mellitus.
In future, we will focus on elucidating the molecular interactions between AGEs and adhesins of the pili present in M. nonliquefaciens to improve our understanding of the pathogenesis of Moraxella keratitis, especially in patients with diabetes. Furthermore, the exploration of therapeutic strategies for inhibiting Moraxella-AGE binding offers novel promising approaches for treatment. Finally, the study of similar bacterial-adhesion mechanisms in other ocular diseases may broaden the scope of this research.
In conclusion, this study offers a fresh understanding of the effects of AGE deposition on bacterial adhesion to the cornea. We have shown in vitro and ex vivo that AGE deposition in the cornea increases the adhesion of M. nonliquefaciens to the cornea. The results suggest that AGE deposition in the cornea of patients with diabetes mellitus is a predisposing factor for developing Moraxella keratitis.
The Institutional Review Board (IRB) of Ehime University approved this study in accordance with the principles of the Declaration of Helsinki (approval number 1503007). Written informed consent was obtained from all patients. The ARRIVE guidelines were followed in performing all methods used in animal tests, which were approved by the Institutional Animal Care and Use Committee of Ehime University (approval number 05HA84-1). Additionally, all the methods were carried out in accordance with relevant Institutional guidelines and regulations.
Clinical and microbiological records of 27 culture-proven Moraxella keratitis cases reported at Ehime University Hospital from March 2006 to February 2022 were retrospectively analyzed in addition to the species and predisposing factors for Moraxella keratitis. The organisms were identified by Gram-stained smears and corneal scrape culture on sheep blood agar at 37 °C and 5% CO2. On a Bruker MALDI Biotyper (Bruker Daltonics, Billerica, MA), MALDI-TOF MS was performed to identify Moraxella species. The identification criteria were a score of ≥ 2.0 for species-level identification.
HCECs immortalized by SV40 were purchased from the RIKEN BioResource Research Center (Ibaraki, Japan) and used. SV40 HCECs were cultured in a supplemental hormonal epithelial medium (SHEM) comprising Dulbecco’s Modified Eagle Medium/F-12 (1:1) from Life Technologies in Grand Island, New York, with 1% antibiotic–antimycotic combination, 0.5 μg/mL hydrocortisone, 0.1 μg/mL cholera toxin, and 0.5% dimethyl sulfoxide in 37 °C humid atmosphere with 5% CO2. Before testing, 24-well plates of HCECs (2.0 × 104 cells/well) were grown to 95% confluence. The concentration of HCECs was determined using an automated cell counter (Countess III automated cell counter; Thermo Fisher Scientific, Waltham, Massachusettes).
A standard strain of M. nonliquefaciens ATCC (American Type Culture Collection) 19,975 was used in each experiment. The strain was raised for 24 h at 37 °C and 5% CO2 in brain–heart infusion broth (Becton Dickinson, Sunnyvale, CA). The bacterial solution was then diluted with phosphate-buffered saline (PBS) to the appropriate concentration. A calibration curve for M. nonliquefaciens (ATCC19975) was constructed to adjust the concentration (Fig. 5). To establish the calibration curve, the turbidity of the bacterial solution was measured using an absorbance meter (CO8000 Biowave Personal Cell Density Meter; Biochrom, Cambridge, UK), and solution was plated onto blood agar for counting the number of colonies.
Calibration curve of Moraxella nonliquefaciens (ATCC19975). y = 0.1043x + 0.0546, R2 = 0.9914.
In this study, 7-week-old male C57BL/6 mice were purchased from CLEA Japan Inc. (Tokyo, Japan). STZ (Sigma-Aldrich, St. Louis, Missouri) (200 mg/kg) was intraperitoneally administered once in 8-week-old male C57BL/6 mice to induce diabetes mellitus in mice55. Diabetic mice with blood glucose levels > 300 mg/dL were used in the experiments. The AGE inhibitor pyridoxamine dihydrochloride (Tokyo Chemical Industry, Tokyo, Japan) in 1 g/L of water was used to suppress AGE deposition in the cornea of diabetic mice. Male C57BL/6 mice began consuming this water 1 week before the intraperitoneal administration of STZ37. We raised these mice until 24 weeks of age and used them for experiments. Diabetic mice (24-week-old), diabetic mice that consumed pyridoxamine (24-week-old), age-matched wild-type mice (24-week-old), and young wild-type mice (8-week-old) were deeply anesthetized with a cocktail of medetomidine hydrochloride (1.0 mg), midazolam (5.0 mg/mL), and butorphanol (5.0 mg/mL). After anesthesia induction, the mice were euthanized by de-bleeding from the axillary artery, and only the cornea was removed and used in the experiments.
An adhesion assay was performed essentially as previously described56,57. The concentrations of AGEs and bacteria were determined based on previous reports36,58. Advanced glycation end product–bovine serum albumin (AGE-BSA) (Cayman Chemical, Ann Arbor, MI) was added to 1 mL of SHEM to achieve a concentration of 100 μg/mL in 10 wells, and 1 mL of SHEM containing BSA was added to another 10 wells in a 24-well plate applied to HCECs (2.0 × 104 cells/well). The plate was incubated at 37 °C in a humid atmosphere with 5% CO2 for 12 h. After incubation, we removed the old medium from each well and added 1 mL of SHEM containing 30 μL of the bacterial solution. The bacterial solution was adjusted to 1.0 × 108 CFU/mL using the calibration curve. To encourage the bacteria to contact the HCECs, the 24-well plates were centrifuged at 100 g for 5 min. They were then incubated at 37 °C with 5% CO2 for 1 h. After incubation, the supernatants were discarded and the HCECs were washed with warm PBS. The HCECs were stripped by immersing them in 150 μL of 0.05% Trypsin–EDTA (Life Technologies, Grand Island, NY) for 5 min, diluted with PBS, and applied to a sheep blood agar. The agar was incubated for 24 h at 37 °C in a humid atmosphere with 5% CO2, and the number of colonies on the agar was counted manually. This test was performed three times.
The corneas removed from diabetic mice, diabetic mice that consumed pyridoxamine, age-matched wild-type mice, and young wild-type mice were fixed in 4% paraformaldehyde for 1 h. Subsequently, 5 µm-thick sections of the corneas were cut. The sections were graded, ethanol-dehydrated, and deparaffinized using xylene. Then, the sections were deparaffinized with xylene, dehydrated with graded ethanol, and immersed in PBS containing 0.2% Triton X on ice for 15 min to activate the antigen. Blocking was accomplished using a Nichirei Histofine SAB-PO (R) kit (Tokyo, Japan). The sections were treated with 10 mg/mL of AGE polyclonal antibody (bs-1158R. Bioss, Boston, MA) in PBS containing 2% BSA and incubated overnight at 4 °C. Streptavidin–biotin-peroxidase and 3,3′-diaminobenzidine tetrahydrochloride were used to visualize immunoreactivity using the Histofine SAB-PO (R) kit (Nichirei, Tokyo, Japan).
Diabetic mice, diabetic mice that consumed pyridoxamine, age-matched wild-type mice, and young wild-type mice were used, with five mice from each group. Six-fold diluted PA-IODO ophthalmic and eye-washing solution (Nihon Tengan Kenkyujyo, Aichi, Japan) and PBS were used to rinse the excised corneas. The corneas were placed in 1 mL of SHEM containing 30 μL of the bacterial solution and incubated for 1 h at 37 °C. The bacterial solution was adjusted to 1.0 × 108 CFU/mL using the calibration curve as that in in vitro experiments. Following PBS rinsing, the corneas were crushed twice using the Micro Smash MS-100 system (Tomy Seiko, Tokyo, Japan) at 825 g for 1 min. The crushed corneas were diluted in PBS and applied to the sheep blood agar. The agar was incubated for 24 h at 37 °C in a humid environment with 5% CO2, and the number of colonies on the agar was counted manually. We performed this experiment three times.
Each experiment was repeated three times. The representative data are shown in the figures. The data were analyzed using JMP statistical software, version 11.2.0 (SAS Institute, Cary, NC), using a two-tailed unpaired t-test. P-values < 0.05 were used to denote statistical significance.
The datasets analyzed during the current study are available from the corresponding author (HI) on reasonable requests.
Eyre, J. W. A clinical and bacteriological study of diplo-bacillary conjunctivitis. J. Pathol. Bacteriol. 6, 1–13 (1900).
Perez Vidakovics, M. L. & Riesbeck, K. Virulence mechanisms of Moraxella in the pathogenesis of infection. Curr. Opin. Infect. Dis. 22, 279–285 (2009).
van den Munckhof, E. H. A. et al. Nasal microbiota dominated by Moraxella spp. Is associated with respiratory health in the elderly population: A case control study. Respir. Res. 21, 181 (2020).
Article PubMed PubMed Central Google Scholar
Hol, C. et al. Moraxella catarrhalis in acute laryngitis: Infection or colonization?. J. Infect. Dis. 174, 636–638 (1996).
Article CAS PubMed Google Scholar
Murphy, T. F., Brauer, A. L., Grant, B. J. & Sethi, S. Moraxella catarrhalis in chronic obstructive pulmonary disease: Burden of disease and immune response. Am. J. Respir. Crit. Care Med. 172, 195–199 (2005).
Article PubMed PubMed Central Google Scholar
Vergison, A. Microbiology of otitis media: A moving target. Vaccine 26(Suppl 7), G5–G10 (2008).
Article PubMed PubMed Central Google Scholar
Kao, C., Szymczak, W. & Munjal, I. Meningitis due to Moraxella nonliquefaciens in a paediatric patient: A case report and review of the literature. JMM Case Rep. 4, e005086 (2017).
Article PubMed PubMed Central Google Scholar
Seal, D. V., Barrett, S. P. & McGill, J. I. Aetiology and treatment of acute bacterial infection of the external eye. Br. J. Ophthalmol. 66, 357–360 (1982).
Article CAS PubMed PubMed Central Google Scholar
Garg, P., Mathur, U., Athmanathan, S. & Rao, G. N. Treatment outcome of Moraxella keratitis: Our experience with 18 cases—A retrospective review. Cornea 18, 176 (1999).
Article CAS PubMed Google Scholar
Berrocal, A. M., Scott, I. U., Miller, D. & Flynn, H. W. Jr. Endophthalmitis caused by Moraxella species. Am. J. Ophthalmol. 132, 788–790 (2001).
Article CAS PubMed Google Scholar
Das, S., Constantinou, M., Daniell, M. & Taylor, H. R. Moraxella keratitis: Predisposing factors and clinical review of 95 cases. Br. J. Ophthalmol. 90, 1236–1238 (2006).
Article CAS PubMed PubMed Central Google Scholar
Inoue, H. et al. Clinical characteristics and bacteriological profile of Moraxella keratitis. Cornea 34, 1105–1109 (2015).
Leung, A. K. C., Hon, K. L., Wong, A. H. C. & Wong, A. S. Bacterial conjunctivitis in childhood: Etiology, clinical manifestations, diagnosis, and management. Recent Pat. Inflamm. Allergy Drug Discov. 12, 120–127 (2018).
Article CAS PubMed Google Scholar
Zafar, H. et al. Clinical characteristics and outcomes of Moraxella keratitis. Cornea 37, 1551–1554 (2018).
Durrani, A. F. et al. Moraxella keratitis: Analysis of risk factors, clinical characteristics, management, and treatment outcomes. Am. J. Ophthalmol. 197, 17–22 (2019).
McSwiney, T. J., Knowles, S. J. & Murphy, C. C. Clinical and microbiological characteristics of Moraxella keratitis. Br. J. Ophthalmol. 103, 1704–1709 (2019).
LaCroce, S. J. et al. Moraxella nonliquefaciens and M. osloensis are important Moraxella species that cause ocular infections. Microorganisms 7, 163 (2019).
Article CAS PubMed PubMed Central Google Scholar
Takahashi, S. et al. Moraxella species: Infectious microbes identified by use of time-of-flight mass spectrometry. Jpn. J. Ophthalmol. 63, 328–336 (2019).
Hoarau, G. et al. Moraxella keratitis: Epidemiology and outcomes. Eur. J. Clin. Microbiol. Infect. Dis. 39, 2317–2325 (2020).
Article CAS PubMed Google Scholar
Kenny , SE , Puig , M. , Salinas , R. , Johnson , DA & Kheirkhah , A. Moraxella keratitis: A case series.Eye Contact Lens 47, 674–676 (2021).
Study Group of National Surveillance of Infectious Keratitis in Japan. National Surveillance of Infectious Keratitis in Japan--current status of isolates, patient background, and treatment. Nippon Ganka Gakkai Zasshi 110, 961–972 (2006).
Puig, M., Weiss, M., Salinas, R., Johnson, D. A. & Kheirkhah, A. Etiology and risk factors for infectious keratitis in South texas. J. Ophthalmic. Vis. Res. https://doi.org/10.18502/jovr.v15i2.6729 (2020).
Article PubMed PubMed Central Google Scholar
Almulhim, A., Alkhalifah, M. I., Kalantan, H. & Alsarhani, W. K. Bacterial keratitis: Clinical features, causative organisms, and outcome during a 13 year study period. Cornea 42, 702–707 (2023).
Schultz, RO, Van Horn, DL, Peters, MA, Klewin, KM & Schutten, WH Diabetic keratopathy.Trans.Am. Ophthalmol.Soc.79, 180–199 (1981).
CAS PubMed PubMed Central Google Scholar
Datiles , MB , Kador , PF , Fukui , HN , Hu , TS & Kinoshita , JH Corneal re-epithelialization in galactosemic rats .Invest.Ophthalmol.Vis.Sci.24, 563–569 (1983).
Huang, SP et al.Aldo-keto reductases in the eye.J. Ophthalmol.2010, 521204 (2010).
PubMed PubMed Central Google Scholar
Kimura, T. et al. Accumulation of advanced glycation end products of the maillard reaction with age in human hippocampal neurons. Neurosci. Lett. 208, 53–56 (1996).
Article CAS PubMed Google Scholar
Singh, R., Barden, A., Mori, T. & Beilin, L. Advanced glycation end-products: A review. Diabetologia 44, 129–146 (2001).
Article CAS PubMed Google Scholar
Kaji, Y. et al. Advanced glycation end products in diabetic corneas. Invest. Ophthalmol. Vis. Sci. 41, 362–368 (2000).
Smuda, M. et al. Comprehensive analysis of maillard protein modifications in human lenses: Effect of age and cataract. Biochemistry 54, 2500–2507 (2015).
Article CAS PubMed Google Scholar
Nagaraj, R. H., Linetsky, M. & Stitt, A. W. The pathogenic role of maillard reaction in the aging eye. Amino Acids 42, 1205–1220 (2012).
Article CAS PubMed Google Scholar
Beisswenger, P. J. et al. Formation of immunochemical advanced glycosylation end products precedes and correlates with early manifestations of renal and retinal disease in diabetes. Diabetes 44, 824–829 (1995).
Article CAS PubMed Google Scholar
Xu, J. et al. Involvement of advanced glycation end products in the pathogenesis of diabetic retinopathy. Cell. Physiol. Biochem. 48, 705–717 (2018).
Article CAS PubMed Google Scholar
Zou, C., Wang, S., Huang, F. & Zhang, Y. A. Advanced glycation end products and ultrastructural changes in corneas of long-term Streptozotocin-induced diabetic monkeys. Cornea 31, 1455–1459 (2012).
Wan, L. et al. The advanced glycation end-products (AGEs)/ROS/NLRP3 inflammasome axis contributes to delayed diabetic corneal wound healing and nerve regeneration. Int. J. Biol. Sci. 18, 809–825 (2022).
Article CAS PubMed PubMed Central Google Scholar
Shi, L., Yu, X., Yang, H. & Wu, X. Advanced glycation end products induce human corneal epithelial cells apoptosis through generation of reactive oxygen species and activation of JNK and p38 MAPK pathways. PLoS One 8, e66781–e66781 (2013).
Article ADS CAS PubMed PubMed Central Google Scholar
Ozer, A. et al. Advanced glycation end products facilitate bacterial adherence in urinary tract infection in diabetic mice. Pathog. Dis. https://doi.org/10.1093/femspd/ftu004 (2014).
Article PubMed PubMed Central Google Scholar
Reid, G. & Sobel, J. D. Bacterial adherence in the pathogenesis of urinary tract infection: A review. Rev. Infect. dis. 9, 470–487 (1987).
Article CAS PubMed Google Scholar
Reid, G., Howard, J. & Gan, B. S. Can bacterial interference prevent infection?. Trends Microbiol. 9, 424–428 (2001).
Article CAS PubMed Google Scholar
Dingle, T. C. & Butler-Wu, S. M. MALDI-TOF mass spectrometry for microorganism identification. Clin. Lab. Med. 33, 589–609 (2013).
Schulthess, B. et al. Evaluation of the Bruker MALDI biotyper for identification of fastidious Gram-negative rods. J. Clin. Microbiol. 54, 543–548 (2016).
Article CAS PubMed PubMed Central Google Scholar
Suto, C., Morinaga, M., Yagi, T., Tsuji, C. & Toshida, H. Conjunctival sac bacterial flora isolated prior to cataract surgery. Infect. Drug. Resist. 5, 37–41 (2012).
PubMed PubMed Central Google Scholar
Reichert, R. & Stern, G. Quantitative Adherence of bacteria to human corneal epithelial cells. Arch. Ophthal. 102, 1394–1395 (1984).
Article CAS PubMed Google Scholar
Reichert, R. W., Das, N. D. & Zam, Z. S. Adherence properties of Pseudomonas pili to epithelial cells of the human cornea. Curr. Eye Res. 2, 289–293 (1982).
Marrs, C. F. & Weir, S. Pili (fimbriae) of Branhamella species. Am. J. Med. 88, 36s–40s (1990).
Article CAS PubMed Google Scholar
Tønjum , T. , Marrs , CF , Rozsa , F. & Bøvre , K. The type 4 pilin of Moraxella nonliquefaciens exhibits unique similarities with the pilins of Neisseria gonorrhoeae and Dichelobacter nodosus (Bacteroides).J. Gen.Microbiol.Rev. 137(2483), 2490 (1991).
Fernández, L. A. & Berenguer, J. Secretion and assembly of regular surface structures in Gram-negative bacteria. FEMS Microbiol. Rev. 24, 21–44 (2000).
Waksman, G. & Hultgren, S. J. Structural biology of the chaperone-usher pathway of pilus biogenesis. Nat. Rev. Microbiol. 7, 765–774 (2009).
Article CAS PubMed PubMed Central Google Scholar
Soto, G. E. & Hultgren, S. J. Bacterial adhesins: Common themes and variations in architecture and assembly. J. Bacteriol. 181, 1059–1071 (1999).
Article CAS PubMed PubMed Central Google Scholar
Monnier, V. M. & Cerami, A. Nonenzymatic browning in vivo: Possible process for aging of long-lived proteins. Science 211, 491–493 (1981).
Article ADS CAS PubMed Google Scholar
Kaji, Y. et al. Advanced glycation end products in Descemet’s membrane and their effect on corneal endothelial cell. Curr. Eye. Res. 23, 469–477 (2001).
Article CAS PubMed Google Scholar
Marion, M. S. & Carlson, E. C. Immunoelectron microscopic analyses of maillard reaction products in bovine anterior lens capsule and Descemet’s membrane. Biochim. Biophys. Acta 1191, 33–42 (1994).
Article CAS PubMed Google Scholar
Metz, T. O., Alderson, N. L., Thorpe, S. R. & Baynes, J. W. Pyridoxamine, an inhibitor of advanced glycation and lipoxidation reactions: A novel therapy for treatment of diabetic complications. Arch. Biochem. Biophys. 419, 41–49 (2003).
Article CAS PubMed Google Scholar
Stitt, A. et al. The AGE inhibitor pyridoxamine inhibits development of retinopathy in experimental diabetes. Diabetes 51, 2826–2832 (2002).
Article CAS PubMed Google Scholar
Wu, K. K. & Huan, Y. Streptozotocin-induced diabetic models in mice and rats. Curr. Protoc. Ppharmacol. 40, 41–45 (2008).
Liang, X. & Ji, Y. Comparative analysis of staphylococcal adhesion and internalization by epithelial cells. Methods Mol. Biol. 391, 145–151 (2007).
Article CAS PubMed Google Scholar
Suzuki, T., Swoboda, J. G., Campbell, J., Walker, S. & Gilmore, M. S. In vitro antimicrobial activity of wall teichoic acid biosynthesis inhibitors against Staphylococcus aureus isolates. Antimicrob. Agents Chemother. 55, 767–774 (2011).
Article CAS PubMed Google Scholar
Suzuki, T. et al. Role of pvdE pyoverdine synthesis in pseudomonas aeruginosa keratitis. Cornea 37, S99–S105 (2018).
We thank Takahiro Fukazawa and Ayame Hyodo for their valuable technical assistance with the experiments. Additionally, we thank Enago (www.enago.jp) for English-language review.
Department of Ophthalmology, Ehime University Graduate School of Medicine, Shitsukawa, Toon, Ehime, 791-0295, Japan
Hidenori Inoue, Koji Toriyama, Naoko Takahira & Atsushi Shiraishi
Clinical Laboratory Division, Ehime University Hospital, Shitsukawa, Toon, Ehime, 791-0295, Japan
Shinobu Murakami & Hitoshi Miyamoto
Department of Ophthalmology, Toho University Graduate School of Medicine, 6-11-1, Omori-nishi, Ota-ku, Tokyo, 143-8541, Japan
You can also search for this author in PubMed Google Scholar
You can also search for this author in PubMed Google Scholar
You can also search for this author in PubMed Google Scholar
You can also search for this author in PubMed Google Scholar
You can also search for this author in PubMed Google Scholar
You can also search for this author in PubMed Google Scholar
You can also search for this author in PubMed Google Scholar
HI, KT, TS, and AS were responsible for study design. HI and KT were contributed to collect and analysis the date. HI, NT, SM, and HM performed the experiments. HI made major contributions to writing the manuscript. HI, KT, and AS were involved in drafting the manuscript and revising it. All authors read and approved the final manuscript.
The authors declare no competing interests.
Springer Nature remains neutral with regard to jurisdictional claims in published maps and institutional affiliations.
Open Access This article is licensed under a Creative Commons Attribution 4.0 International License, which permits use, sharing, adaptation, distribution and reproduction in any medium or format, as long as you give appropriate credit to the original author(s) and the source, provide a link to the Creative Commons licence, and indicate if changes were made. The images or other third party material in this article are included in the article's Creative Commons licence, unless indicated otherwise in a credit line to the material. If material is not included in the article's Creative Commons licence and your intended use is not permitted by statutory regulation or exceeds the permitted use, you will need to obtain permission directly from the copyright holder. To view a copy of this licence, visit http://creativecommons.org/licenses/by/4.0/.
Inoue, H., Toriyama, K., Takahira, N. et al. Association between Moraxella keratitis and advanced glycation end products. Sci Rep 14, 8024 (2024). https://doi.org/10.1038/s41598-024-58659-7
DOI: https://doi.org/10.1038/s41598-024-58659-7
Anyone you share the following link with will be able to read this content:
Sorry, a shareable link is not currently available for this article.
Provided by the Springer Nature SharedIt content-sharing initiative
By submitting a comment you agree to abide by our Terms and Community Guidelines. If you find something abusive or that does not comply with our terms or guidelines please flag it as inappropriate.
Scientific Reports (Sci Rep) ISSN 2045-2322 (online)

What Is An Independent Distributor Sign up for the Nature Briefing newsletter — what matters in science, free to your inbox daily.