Over the years, scientific studies have not conclusively shown that [zinc or vitamin C] can help overcome illnesses such as the common cold. Since the pandemic began, however, both supplements have seen an increased market owing to people’s belief that they can give the immune system a boost. The New York Times reported zinc sales of $134 million, and USA Today found that vitamin C sales reached $209 million during the first half of 2020, up 76% compared with 2019.
To see how zinc and ascorbic acid could affect COVID-19, the researchers randomly assigned 10 days of zinc gluconate (50 milligrams per day), ascorbic acid (8,000 milligrams per day), both, or standard of care to 58, 58, 48, and 50 people, respectively. Outpatients knew which treatment they were receiving. 1h,1h,2h,2h-Heptadecafluorodecyl Acrylate
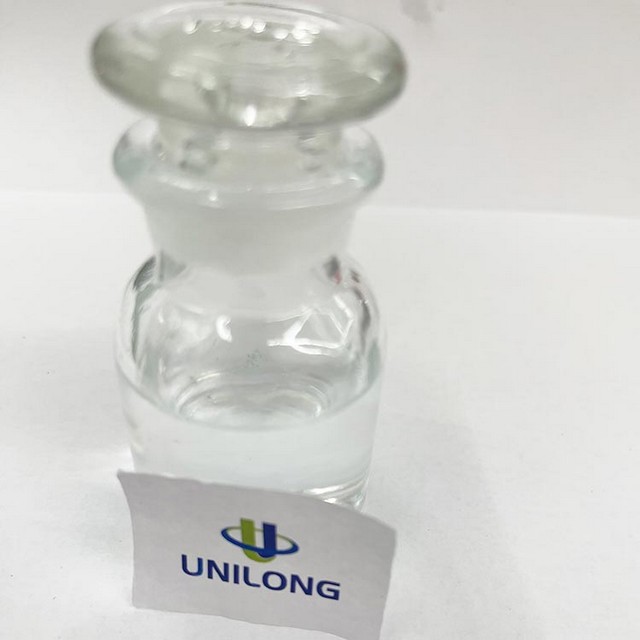
The study ended early because it met the futility criteria, as assessed by Cleveland Clinic’s operational and safety monitoring board. “These interventions did not significantly shorten the duration of symptoms associated with the virus compared with usual care,” the researchers conclude.
Follow the latest news and policy debates on sustainable agriculture, biomedicine, and other ‘disruptive’ innovations. Subscribe to our newsletter. SIGN UP

Dipentene The researchers also looked at hospitalization rate, mortality, and adverse effects from receiving one of the treatments or standard of care. No arm of the experiment had significantly different outcomes, but the researchers note that those who received ascorbic acid reported slightly more nausea, diarrhea, and stomach cramps.