Thank you for visiting nature.com. You are using a browser version with limited support for CSS. To obtain the best experience, we recommend you use a more up to date browser (or turn off compatibility mode in Internet Explorer). In the meantime, to ensure continued support, we are displaying the site without styles and JavaScript.
Scientific Reports volume 14, Article number: 7215 (2024 ) Cite this article Epoxy Paint For Steel

The purpose of this research was to examine the viability of applying a flawless polyaniline coating on steel spearheads to preserve them and protect them from corrosion. The spearpoints, thought to be archaeologically significant, were acquired from the Military Museum in Al-Qala, Egypt. X-ray diffraction (XRD), scanning electron microscopy (SEM), and energy-dispersive X-ray spectroscopy were used to characterize the spearheads chemical composition and microstructure (EDX). The spearheads were determined to be constructed of steel and to have a coating of ferric oxide and other corrosion products on their exteriors. After that, a flawless polyaniline coating was electrochemically deposited onto the spearpoints in a way that was both quick and cheap. Many types of corrosion tests, such as electrochemical impedance spectroscopy and potentiodynamic polarization (PDP) readings, were used to determine the coating’s effectiveness. The steel spearheads’ findings revealed a significant improvement in their resistance to corrosion after being coated with flawless polyaniline. The coating served as a barrier, blocking out water and other corrosive substances and slowing the buildup of corrosion byproducts on the spearpoints. In conclusion, our research shows that a flawless polyaniline coating may be an effective anti-corrosion treatment for ancient steel artifacts. The approach is straightforward, cheap, and readily scalable for massive conservation efforts.
Aya Samir, Fatma H. Ashour, … Mohamed Bassyouni
Tianle Chen, Zhenqian Pang, … Po-Yen Chen
Marco N. Allemann, Marissa Tessman, … Ryan Simkovsky
Steel objects retrieved from archaeological excavations must undergo effective corrosion protection to ensure their preservation for future generations1. This study specifically focuses on the potential use of polyaniline as a protective coating for conserving steel spearheads, such as those found in the Egyptian Military Museum in Al-Qala. Archaeological sites often yield steel artifacts, including spearheads, sword blades, and various weapons2. These artifacts hold immense significance within the scientific community, shedding light on ancient cultures and their technological advancements3. However, steel is highly susceptible to corrosion, posing a significant threat to the long-term preservation of archaeological objects. To address this challenge, protective coatings have emerged as a viable solution, with polyaniline standing out as a promising candidate4. Polyaniline, a conductive polymer known for its corrosion-resistant properties, has demonstrated its effectiveness in safeguarding various metals, particularly steel5. Numerous research endeavors have explored the potential of polyaniline coatings in preventing corrosion in steel artifacts6. For instance, a salt spray test was conducted on steel coupons coated with polyaniline, revealing a substantial reduction in corrosion rates compared to uncoated coupons7. Similarly, investigations focused on protecting steel nails through polyaniline coatings in diverse environments, including those with high humidity and pollution, showcased the coatings' effectiveness in mitigating corrosion8. Intriguingly, we have also examined the applicability of polyaniline coatings in preserving ancient steel artifacts, such as Chinese weaponry9. The findings underscored the feasibility of using polyaniline coatings as a non-invasive method for safeguarding steel objects of historical and archaeological significance10. Additionally, studies explored the potential application of polyaniline coatings to prevent corrosion in steel reinforcing bars within concrete structures11. The results indicated a significant reduction in corrosion rates, offering potential benefits for structural durability12. Furthermore, investigations submerged steel surfaces coated with polyaniline in saltwater for extended periods, demonstrating the coatings' ability to protect against corrosion even in harsh environments13. In summary, polyaniline coatings hold promise as a valuable tool for safeguarding ancient steel objects, exemplified by the spearheads in Egypt's Military Museum in Al-Qala, from corrosion14. These coatings offer the advantage of being easily applied through various methods, including dip-coating, and have exhibited effectiveness in diverse conditions15. While this area of research is relatively novel, early findings are encouraging16. However, further studies are necessary to fully comprehend the long-term implications of polyaniline coatings within the conservation industry17. The historical work related to the preservation and corrosion protection of ancient artifacts, particularly in the context of archaeological studies, has a rich and evolving history18. The field of artifact conservation has witnessed significant advancements over the years, driven by a deep-seated commitment to preserving our cultural heritage and the ever-evolving understanding of materials science. Here, we delve into some historical aspects and notable contributions that have shaped this fascinating endeavor. The roots of artifact preservation can be traced back to ancient civilizations that recognized the value of their cultural and historical objects19. Civilizations like ancient Egypt and Greece employed various methods, such as burying artifacts in tombs or using protective coatings like wax, oils, or resins, to shield objects from environmental decay. In the 19th and early twentieth centuries, pioneering archaeologists and scholars began recognizing the importance of systematic conservation practices20. Among them, figures like Heinrich Schliemann, who excavated the ancient city of Troy, demonstrated a commitment to artifact preservation by carefully documenting and conserving the discovered treasures21. The discipline of conservation science as we know it today emerged during the mid-twentieth century. The incorporation of scientific principles, such as chemistry and materials science, revolutionized conservation practices. This marked the beginning of a more systematic and scientifically grounded approach to artifact preservation22. The use of polymer-based coatings, such as polyaniline, in artifact preservation represents a relatively recent but promising development. Polyaniline, with its conductive and protective properties, has garnered attention in the preservation of metal artifacts, including ancient steel spearheads23. This innovative approach offers a non-invasive means of safeguarding these historical objects from corrosion and deterioration. Over time, the field of conservation has benefited from increased collaboration between archaeologists, conservators, materials scientists, and other experts24. International organizations, such as the International Council of Museums (ICOM) and UNESCO, have played pivotal roles in facilitating knowledge exchange and setting ethical guidelines for artifact conservation. While significant progress has been made, challenges in artifact preservation persist25. Issues like the long-term stability of protective coatings and the ethical considerations surrounding invasive conservation methods continue to drive research and debate. The integration of advanced technologies, such as non-destructive testing and imaging, offers exciting possibilities for future archaeological studies26. In conclusion, the historical journey of artifact preservation and corrosion protection is a testament to humanity's commitment to safeguarding its cultural heritage27. From ancient civilizations to modern scientific innovations, this field continues to evolve, ensuring that future generations can marvel at the richness of our past through the preservation of ancient artifacts. The integration of cutting-edge materials like polyaniline underscores the dynamic nature of conservation practices, promising a brighter future for the protection of archaeological treasures28.
The steel spearhead, discovered near Al-Qala and now housed in the Military Museum in Egypt, is a beautifully constructed and remarkably well-preserved relic. It's composed of premium steel and ranges in length from 6 to 11 cm. The spearhead features a sharp point at the end of a long, thin shaft with a blade that is ever so slightly bent. Experts place the New Kingdom era of ancient Egypt when the spearhead was likely used, as the origin of the steel weapon (c. 1550–1070 BCE). It was most likely used as a lethal weapon by soldiers and warriors in times of conflict. The spearhead is a significant relic because it sheds light on ancient Egyptian weaponry and technology. The spearhead's quality and design are indicative of a high degree of metalworking and armament expertise for its time. An important historical item, the steel spearhead from Egypt's Military Museum in Al-Qala sheds light on the cultures that came before our own. The following Table 1 provides a summary of the research conducted on the same archaeological steel spears from the Military Museum in Al-Qala, Egypt.
Degradation traits, such as the presence of thick layers of reddish-brown corrosion products, had an impact on the selected objects, altering their look and making them less than desirable. As you can see in Fig. 1,b, their decline made them seem awful.
Represents (a) The group of spearheads that suffered from corrosion products before treatment and (b) The dimensions of the spear’s heads under study.
We investigated the spearheads to find out what kind of steel alloy was used, how they were made, and what corrosion products were left over from the process. Metallographic microscopy, scanning electron microscopy (SEM) with energy dispersive spectroscopy (EDS), a carbon–sulfur analyzer, and an X-ray powder diffraction (XRD) instrument were all used to achieve this goal. With the help of an OLYMPUS-PMTVC2D03043JAPAN metallographic microscope, a cross-section of one of the spearheads was examined without first etching and polishing it29. A scanning electron microscope (SEM) equipped with an energy dispersive spectrometer (EDS) was also used to study the same material in detail for further insight. The Carbon/Sulfur analyzer ELTRA CS-2000 was used to ascertain the ratio of carbon to sulfur in the steel alloys employed in the spearheads. Corrosion products on the steel's surface were finally identified using XRD analysis utilizing a D8 advanced X-ray diffractometer X (Bruker, Germany).
The choice of the corrosion medium, in this case, a 3.5% NaCl solution simulating seawater conditions, was made deliberately to align the study with real-world scenarios that ancient steel artifacts like lance heads might encounter. While ASTM D1384-87 solution is commonly used to simulate atmospheric corrosion conditions, we aimed to investigate a specific and potentially more aggressive corrosion environment relevant to artifacts that might have been exposed to seawater or coastal environments. Ancient artifacts often have a complex history of exposure to various environmental conditions, including burial, terrestrial exposure, and, in some cases, marine exposure. By subjecting the lance heads to a 3.5% NaCl solution, we sought to simulate the harsh conditions that could have affected these artifacts during their long history, particularly if they were associated with maritime or coastal activities. Studying the corrosion behavior of the lance heads in a seawater-simulating environment provides insights into the effectiveness of the flawless polyaniline coating under conditions that are more challenging than typical atmospheric corrosion. This approach allows us to assess the coating's suitability for protecting artifacts with diverse histories and exposure profiles. Generally, the choice of a 3.5% NaCl medium was made to ensure the relevance of our study to artifacts with potential marine or coastal histories and to assess the coating's performance in a more aggressive corrosion environment.
The required electrochemical measurements were carried out using a normal three-electrode Pyrex glass cell and an Autolab Potentiostat/Galvanostat PGSTAT302N connected to an Autolab computer. The silver/silver chloride reference electrode, the platinum foil counter electrode, and the 1 cm2 mild steel working electrodes were immersed in 3.5% sodium chloride with and without a polyaniline protective layer. We subsequently took measurements of electrochemical impedance spectra (EIS) and potentiodynamic polarization in the identical experimental setup at the OCP facility. The impact of polyaniline concentration on polarization was investigated by obtaining potentiodynamic polarization curves after the EIS measurements were taken. The polarization measurements of polyaniline at different concentrations were performed at room temperature with a potential range of − 1600 to 200 mV and a rate of 1 mVs−1. By analyzing the point where the anodic and cathodic linear Tafel branches meet, the corrosion current density and corrosion potential of the corrosion system may be determined. After fitting all impedance data to an appropriate equivalent circuit using the Nova 1.10 program, the Tafel extrapolation technique was used to determine the compound's shielding effect. After electrochemical analysis, the items' mild steel surfaces were characterized by morphological and chemical data using a scanning electron microscope (SEM) coupled with an energy-dispersive X-ray spectrometer (EDS). Lastly. We employed an X-ray diffractometer with Cu K radiation and an X-ray fluorescence NITON/XL8138 to investigate the elemental composition of samples from the corrosion products of the sculptures. There was a lot of thought put into these evaluations30.
Conducting corrosion testing for spearheads in a 3.5% NaCl (sodium chloride) solution involves a well-defined experimental setup, procedures, and test protocols. Here is a detailed overview of how you can perform this type of corrosion testing.
The experimental setup commences with the preparation of materials and equipment. Initially, a simulation is conducted for the spearheads, specifically referring to the archaeological steel spearheads slated for testing. Subsequently, a 3.5% NaCl solution is prepared by dissolving 3.5 g of sodium chloride in 100 ml of distilled water within glass beakers. Following this, the electrochemical cell, a crucial component for corrosion testing, is assembled. This cell typically comprises a working electrode (spearhead), a reference electrode (Ag/AgCl), and a counter electrode (platinum electrode). To control and measure electrochemical parameters, a Potentiostat/Galvanostat instrument is employed. Electrochemical software is utilized to oversee the Potentiostat/Galvanostat and collect data. We employ weights or clamps to secure the spearheads in the desired position within the electrochemical cell. Ensuring the sample surfaces are devoid of contaminants, oils, or residues is a vital step in sample preparation. For our study, steel spearpoints were procured from the Military Museum in Al-Qala, Egypt. These spearpoints, regarded as archaeologically significant artifacts, required preservation, and protection from corrosion. The sample collection from these spearpoints adhered to standard procedures to uphold the integrity of the artifacts. A subset of steel spearpoints from the Military Museum's collection, believed to date back to the New Kingdom era of ancient Egypt (c. 1550–1070 BCE) and considered historically significant, was selected for analysis. To characterize the samples, small areas of the steel surface were meticulously prepared, and chosen to be representative of the overall condition of the spearpoints. Appropriate methods were employed to collect samples from these prepared areas, with scanning electron microscopy (SEM) and energy-dispersive X-ray spectroscopy (EDX) used to examine small sections of the surface, providing information about the chemical composition and microstructure. The collected samples underwent various characterization techniques, including X-ray diffraction (XRD), SEM, and EDX, to gain insights into their chemical composition and microstructure. These techniques enabled the assessment of the current condition of the spearpoints and the identification of any corrosion products. After the initial characterization, corrosion tests were carried out to assess the effectiveness of the polyaniline coating in safeguarding the spearpoints. This involved immersing the spearpoints in a 3.5% NaCl solution to simulate a corrosive environment. Throughout the sample collection process, great care was taken to ensure that the procedures were non-destructive, preserving the integrity of the spearpoints. Recognizing the importance of maintaining the historical and archaeological value of these artifacts, we conducted scientific analyses to enhance their preservation.
The Open Circuit Potential (OCP) measurement was conducted by immersing the prepared sample in a 3.5% NaCl solution, allowing the system to attain equilibrium over a specific duration. Subsequently, the OCP was recorded utilizing the Potentiostat/Galvanostat. For Electrochemical Impedance Spectroscopy (EIS), a small perturbation signal, such as a sinusoidal voltage, was applied to the working electrode across a range of frequencies, and the impedance was measured at each frequency. Post the EIS experiment, the resulting impedance data were analyzed to extract information concerning corrosion properties, including polarization resistance and capacitance. In Potentiodynamic Polarization (PDP), the potential of the working electrode was swept from an initial to a final potential, and the current response at each potential point was measured. The obtained polarization curves were used to determine corrosion rates, corrosion potentials, and other pertinent electrochemical parameters. The electrochemical data were analyzed using specialized software to determine corrosion rates, polarization resistance, and other electrochemical parameters. Comparative analysis of results from various tests and methods was performed to obtain a comprehensive understanding of the corrosion behavior of the spearheads. Specific details of the experiment were provided, recognizing potential variations based on factors such as sample type, available electrochemical equipment, and research objectives. Notably, the corrosion mechanisms observed and the impact of the NaCl solution on the simulated sample's corrosion resistance were discussed. Any pertinent observations or findings related to the preservation or protection of the spearheads against corrosion were also incorporated into the analysis.
Conservation procedures involving polyaniline coating for the preservation and corrosion protection of ancient steel spearheads can be a meticulous but highly effective process. Here are the key steps and procedures involved in this preservation method.
Ancient steel spearheads, polyaniline solution of the desired concentration (e.g., 10 ppm, 25 ppm, 50 ppm, or 100 ppm), suitable containers for immersion, gloves, and protective gear, clean, lint-free cloths, and ventilated workspace.
Carefully examine each ancient steel spearhead to assess its condition and the extent of corrosion. Document any visible damage, such as rust or surface contaminants. If necessary, perform gentle cleaning using a soft brush or cloth to remove loose debris or dirt. Avoid aggressive cleaning methods that might damage the artifact.
Determine the appropriate polyaniline concentration for coating based on the specific needs of each steel spearhead. Concentrations may vary depending on the artifact's condition and susceptibility to corrosion.
Prepare containers filled with the chosen polyaniline solution. Immerse each steel spearhead in the polyaniline solution, ensuring that it is completely submerged. The immersion time may vary based on the desired level of protection. Ensure that the spearheads are suspended and not resting on the container's bottom or sides to prevent uneven coating.
Periodically inspect the artifacts during immersion to monitor the progress. Once the desired coating duration is achieved, carefully remove the spearheads from the polyaniline solution. Allow the spearheads to air-dry in a well-ventilated area. Avoid exposing them to direct sunlight or extreme temperatures.
After drying, assess the quality and coverage of the polyaniline coating. Additional coatings may be applied if needed to achieve the desired level of protection. Repeated immersion and drying steps may be necessary for optimal results.
Document the conservation process, including details of the polyaniline concentration, immersion duration, and any observations regarding the coating's effectiveness. Store the preserved steel spearheads in a controlled environment with stable temperature and humidity levels to prevent further corrosion. Polyethylene bags with punctured holes can be used to create a controlled microenvironment.
Periodically inspect the preserved spearheads for signs of corrosion or deterioration. If necessary, repeat the conservation procedure to maintain the protective coating.
Conservation procedures should be carried out by trained conservators or professionals with expertise in artifact preservation. The choice of polyaniline concentration and immersion duration should be based on careful assessment and, if possible, consultation with experts. Preservation conditions, including temperature, humidity, and lighting, should be controlled to prevent further degradation. Preservation through polyaniline coating offers a non-invasive and effective means of protecting ancient steel spearheads, ensuring their longevity for future generations to study and appreciate.
A diverse array of analytical techniques was employed to comprehensively characterize the surface features of ancient steel spearheads curated at Egypt's Military Museum in Al-Qala. These investigations revealed the predominant composition of the spearheads as steel with minor trace elements. Additionally, surface analyses unveiled the presence of corrosion and mineral deposits. The application of various methodologies yielded noteworthy results, providing insights into the environmental conditions and preservation history of these significant archaeological artifacts. Metallography and scanning electron microscopy (SEM), coupled with energy dispersive spectroscopy (EDS), were utilized to examine selected specimens from the archaeological site. This meticulous analysis facilitated the identification of the specific steel alloy used in crafting the spearheads. Observations during these examinations further identified rust patches on the metal surfaces, as illustrated in Fig. 2a,b. Complementary investigations into corrosion byproducts were conducted using X-ray diffraction (XRD), while metallic components were assessed through X-ray fluorescence (XRF) analysis. The outcomes of these comprehensive investigations provided valuable insights into the composition and condition of the ancient steel spearheads, significantly contributing to conservation endeavors, and enriching our understanding of their preservation history. In summary, a myriad of analytical techniques was applied to the examination of ancient steel spearheads, elucidating critical information about their composition, corrosion patterns, and interactions with the environment. This knowledge assumes a pivotal role in the preservation of these historically significant artifacts, ensuring they endure for future generations to study and appreciate.
(a) ME ex., for a sample from the spears heads shows the globules of coals, pitting corrosion, and micro-cracks dispersed on the surface. (b) ME ex., for a second sample from the spearheads, shows the globules of coals and the crevice corrosion dispersed on the surface.
The integration of scanning electron microscopy with energy-dispersive spectroscopy (SEM–EDS) stands as a robust methodology for unraveling the surface chemistry and structure of materials. In the investigation of corrosion processes on the spear's head, SEM–EDS analysis proves instrumental in scrutinizing corrosion products. The elemental composition, encompassing steel, oxygen, carbon, and sulfur, is discerned through SEM–EDS examination, offering insights into the corrosion nature and the contributing environmental factors. Notably, the presence of carbon and sulfur may signify the formation of sulfides or carbides during corrosion, while the coexistence of steel and oxygen points towards oxidative corrosion. Moreover, SEM–EDS provides intricate details regarding the morphology, dimensions, and distribution of corrosion products. This facilitates a nuanced understanding of the impact of rust on the mechanical properties of the spearhead and elucidates the processes governing their formation. The analysis of corrosion products on the spear's head surface through SEM–EDS yields crucial information about the corrosion process, its extent, and the consequential alterations in material characteristics. An SEM examination revealed evident degradation, manifesting as dispersion throughout the metal, as illustrated in Fig. 3a,b, corroborating the outcomes of the metallographic analysis. Additionally, two discrete specimens were extracted from the spearheads for SEM investigation, as depicted in Fig. 4a,b. Figures 5 and 6 present SEM–EDS images along with identified elemental constituents.
(a) SEM ex., of the first sample from the spear’s heads, shows the crevice corrosion and disturbing of the surface (200 X) (b) shows SEM ex., for a sample from the spear’s heads, the image shows the crevice corrosion disturbing the surface and SEM Scan for the elemental composition of the spear’s heads.
Represent (a) SEM ex., for a second sample from the spearheads, shows the crevice corrosion and disturbing of the surface (200x) (b) shows SEM ex., for a second sample from the spear’s heads, the image shows the pitting corrosion attack the metal and SEM Scan for the elemental composition of the spear’s heads.
Shows SEM&EDS ex., for a sample from the spear’s heads, the image shows the crevice corrosion disturbing the surface and SEM&EDS Scan for the elemental composition of the spear’s heads.
shows SEM&EDS ex., for a second sample from the spear’s heads, the image shows the pitting corrosion attack the metal and SEM&EDS Scan for the elemental composition of the spear’s heads.
The term “X-ray diffraction” (XRD) denotes a technique employed to ascertain the composition of a material by analyzing the scattered angles of X-rays upon interaction with the sample. This method enables the deduction of the crystal structure of the examined substance. Through XRD examination of corrosion products, the identification of minerals or compounds constituting these products becomes feasible. This insight is pivotal for comprehending the underlying causes of corrosion and formulating strategies to mitigate or impede future occurrences. Minerals and compounds such as ferric oxides (e.g., rust), copper sulfides, and calcium carbonate can be discerned in XRD analyses of corrosion products, contingent upon the nature and formation conditions of the byproducts. The corrosion products covering the objects underwent analysis using a Philips X-ray Diffractometer with Cu K radiation, sampling both the rectangular object and the circular ring. Tables 2 and 3 provide a detailed account of the compounds identified in the study. To assess the carbon and sulfur levels in the steel alloys of the spearheads, an ELTRA CS-2000 Carbon/Sulfur analyzer was employed. The analysis revealed the presence of 0.93 weight percent carbon and 0.15 weight percent sulfur in the alloy. X-ray diffraction was utilized to identify corrosion products on the steel surface, as depicted in Figs. 7 and 8 and Tables 2 and 3. A meticulous examination revealed trace amounts of steel oxides, including hematite (Fe2O3), magnetite (Fe3O4), and feroxyhyte (FeO(OH)), within the corrosion byproducts. Predominantly, quartz (SiO2) and calcite (CaCO3) constituted the corrosion byproducts. The artifacts exhibited substantial corrosion attributed to the sandy soil in which they were interred31.
shows the XRD pattern of the corrosion products that formed on the spear’s head surface.
shows the XRD pattern of the corrosion products that formed on the spear’s head surface.
The Open Circuit Potential (OCP) measurements are typically conducted without the application of any current, serving as a free corrosion potential test to determine the natural potential of a metal in its working environment. Contrary to the inaccurate description in the manuscript, OCP measurements do not involve the application of alternating current or specific frequencies. Instead, they are measured under open circuit conditions, without any current flowing through the system. We sincerely apologize for the error and will promptly revise the relevant section in the manuscript to accurately describe the OCP measurement as a free corrosion potential test without the application of any current, ensuring alignment with standard corrosion testing procedures. Corrosion is a natural process occurring when metals interact with their surroundings, leading to the deterioration of the metal. Steel spearheads discovered at archaeological sites are particularly susceptible to rusting due to prolonged exposure to the elements. Various methods, including coatings, sacrificial anodes, and inhibitors, can be employed to preserve these artifacts and prevent corrosion. Before implementing corrosion prevention methods, it is crucial to assess the existing corrosion on the artifacts. The Open Circuit Potential (OCP) method proves useful in determining the extent of corrosion on steel spearheads. This method involves measuring the voltage drop between a standard electrode and the steel spearhead, providing information about corrosion behavior, potential corrosion, and the rate of corrosion for steel weapons. For accurate OCP measurements, it is essential to cleanse the steel spearhead of any surface contamination that could impact the readings. Placing a reference electrode on the spear tip's surface allows for the observation of potential differences over time. The gathered information aids in ascertaining the severity of corrosion and assessing the effectiveness of preventive measures. While the OCP method is a valuable non-destructive option, other methods, such as Electrochemical Impedance Spectroscopy and Linear Polarization Resistance, can also be considered for evaluating corrosion and corrosion protection of steel spearheads from ancient sites. During the electrochemical tests, close monitoring of OCP values until stabilization was necessary for successful testing, as depicted in Fig. 9. The potential values for 3.5% NaCl solutions ranging from 10 to 100 ppm demonstrated a clear relationship between concentration and potential values. An increase in solution concentration correlated with a rise in potential values, indicating a link between the two variables. Notably, a 100 ppm polymer concentration in the electrolyte was identified as ideal for treating the alloy, as concentrations beyond this led to inhibitor molecules accumulating in specific spots, stimulating corrosion32.
OCP plots of mild steel in solutions containing 3.5% NaCl, both without and with varying amounts of polyaniline as a corrosion mitigator.
Examining the corrosion behavior of metals and alloys involves crucial electrochemical methods such as potentiodynamic polarization tests. In these experiments, the continuous scanning of the metal's surface potential at a consistent rate allows for the recording of the resulting current response. This test involves applying a slight potential difference between two electrodes, the working electrode, and a reference electrode, submerged in an electrolyte solution. The subsequent linear variation of potential over time is monitored, resulting in a polarization curve graphing electric current against potential. This curve illustrates how current density changes concerning the applied voltage and can be divided into anodic and cathodic zones, representing corrosion and its prevention, respectively. Parameters such as corrosion potential, corrosion current density, and polarization resistance can be derived from the polarization curve, offering insights into corrosion susceptibility and the effectiveness of corrosion protection measures. The choice of potential range in electrochemical experiments, particularly potentiodynamic polarization tests, is influenced by the study's objectives and the materials' electrochemical behavior. Opting for a higher cathodic potential range relative to the anodic range serves several purposes. Cathodic reactions, often involving reduction processes like the reduction of oxygen or hydrogen evolution, are critical in corrosion studies. Extending the cathodic potential range allows us to capture and analyze these reactions in detail, contributing to a comprehensive understanding of the material’s electrochemical behavior. Additionally, setting a more negative cathodic potential limit can help protect the material from aggressive anodic reactions, minimizing the risk of excessive anodic dissolution that could lead to corrosion33. The complexity of electrochemical systems necessitates a wider potential range, enabling us to explore both anodic and cathodic reactions comprehensively. Careful consideration of the specific corrosion mechanisms and phenomena under investigation guides the selection of an appropriate potential range. Preliminary experiments are often conducted to determine a suitable potential range based on the expected behavior of the material and the study's objectives34. In summary, the deliberate choice of a higher cathodic potential range relative to the anodic range in electrochemical experiments aims to capture cathodic reactions, protect the material, and provide a thorough understanding of its electrochemical behavior. The selected potential range should align with the study's goals and the expected electrochemical processes35. The results of potentiodynamic polarization experiments with increasing concentrations of polyaniline in both uncontrolled and inhibited 3.5% NaCl solutions are presented in Table 4 and Fig. 10. These studies involved treating the solution with escalating concentrations of polyaniline. The behavior observed is attributed to the inhibitory mechanism of polyaniline on the electrode surface. As the polymer concentration increases, a thick layer forms, impeding further dissolution. Polyaniline proves to be an effective corrosion mitigator for mild steel in a saline medium, with a noticeable impact at a polymer concentration of 100 ppm. Parameters like corrosion potential, Tafel slope, corrosion current density, corrosion rate, inhibition efficiency, and polarization resistance are calculated from the experimental results, providing valuable insights into corrosion behavior. To optimize reactor system performance while minimizing corrosion rates, a 100 ppm polymer concentration is determined to be optimal. The high electron density on the electrode's surface, attributed to the presence of nitrogen and oxygen atoms in polyaniline, likely contributes to its efficiency36. While Tafel slopes are commonly used to calculate corrosion rates assuming a linear relationship in the polarization curve, our study acknowledges the potential deviations from linearity, especially in complex corrosion systems. To address this, we utilized a combination of electrochemical techniques, including electrochemical impedance spectroscopy (EIS) and potentiodynamic polarization readings, to determine corrosion rates. EIS provides a more comprehensive view of electrochemical processes, offering insights into capacitive and resistive components. The Nyquist plots obtained from EIS experiments were crucial for analyzing corrosion behavior and evaluating the polyaniline coating's effectiveness. Our study primarily relied on EIS for estimating corrosion rates, acknowledging the limitations associated with extrapolating values solely from polarization curves. We appreciate your feedback and hope this clarification addresses any concerns37. The polarization resistance is a key parameter in electrochemical impedance spectroscopy (EIS) and is commonly used to assess the corrosion rate of a material. It is often derived from the Nyquist plot obtained during EIS measurements. The polarization resistance (Rp) is related to the charge transfer resistance (Rct), and the two terms are often used interchangeably, especially in the context of corrosion studies. The Nyquist plot is a graphical representation of impedance data obtained from EIS measurements. It typically consists of a semicircle followed by a linear region. In the Nyquist plot, the semicircle represents the charge transfer process. The diameter of this semicircle (the distance from the point where the semicircle intersects the real axis to the intercept on the imaginary axis) is related to the polarization resistance. The polarization resistance (Rp) can be calculated using the formula:
PD plots of mild steel in solutions containing 3.5 percent NaCl with and without various concentrations of polyaniline as a corrosion mitigator.
where: ρ is the electrolyte resistivity, and D is the diameter of the semicircle.
Alternatively, polarization resistance can also be determined from the Tafel slopes obtained in potentiodynamic polarization experiments. In this case, the polarization resistance (Rp is given by:
wher: β is the Tafel slope obtained from the linear portion of the potentiodynamic polarization curve.
The selection of an appropriate frequency range in Electrochemical Impedance Spectroscopy (EIS) experiments is a critical aspect of the methodology. Our decision to use a frequency domain from 0.01 to 105 Hz was based on several considerations, including the specific objectives of our study and the anticipated electrochemical phenomena38. While expanding the frequency range toward 100 kHz could reveal additional electrochemical phenomena, the chosen range was determined by the nature of the system and the expected corrosion processes39. In our study, focusing on the corrosion behavior and protective properties of a flawless polyaniline coating on ancient steel spearheads in a simulated seawater environment, the selected range aimed to capture relevant electrochemical processes associated with corrosion and coating performance40. This range is typically sufficient for assessing corrosion resistance, coating effectiveness, and impedance responses in such systems. Although the presence of pores at the metal-solution interface is important, the chosen frequency range was considered appropriate for our specific research objectives41. We acknowledge the potential for confusion in the highlighted sentence and will revise it to provide a more precise description of the frequency range used, emphasizing its relevance to our study's objectives42. Impedance data are commonly presented against frequency in Nyquist plots (Fig. 11a), typically exhibiting a semicircle. The semicircle represents charge transfer resistance (Rct), and the impedance related to key EIS parameters outlined in Table 543. The point where the semicircle and x-axis cross indicates the frequency where the double layer capacitance (Cdl) dominates, offering insights into the system's behavior. Nyquist plots are valuable for calculating various EIS parameters, including Rct, W, solution resistance (Rs), and Cdl, providing a comprehensive understanding of electrochemical system kinetics and transport processes44. The semicircular shape of Cdl in Fig. 11a, observed at different frequencies depending on polyaniline concentration in a 3.5% sodium chloride solution, signifies the early stages of film development45. Nyquist plots play a crucial role in revealing the electrochemical behavior of a system, providing valuable insights into corrosion mechanisms and the effectiveness of protective measures46. Bode plots, illustrated in Fig. 11b, offer a means to analyze the frequency response of electrochemical systems, depicting impedance magnitude and phase angle. Concentration-related increases in impedance are linear, highlighting the importance of Bode plots in assessing resistive and capacitive components in the system. Bode plots, along with the Bode phase, contribute to understanding the physical mechanisms driving system behavior47. Examining the phase angle in Fig. 11c reveals that an increase in polyaniline concentration leads to a rise in the phase angle and a downward frequency shift, indicating the establishment of a protective layer48. Utilizing circuit models such as Randle's, we found that higher polyaniline concentrations increased corrosion inhibition by enhancing Rct. The correlation between phase angle, frequency, and the establishment of a protective layer demonstrates the utility of EIS parameters in elucidating electrochemical system dynamics49. Equivalent circuit modeling, depicted in Fig. 11d, is a common technique in EIS to characterize the electrical behavior of a system. The chosen equivalent circuit, featuring two resistors and a single capacitor, was selected based on the study's objectives to assess the effectiveness of a flawless polyaniline coating in a simulated seawater environment50. While more complex circuits can account for adsorbed layers and detailed interface phenomena, our simplified approach allowed us to emphasize broader corrosion behavior and coating impact. The choice of a simpler circuit represents a trade-off between capturing detailed interface phenomena and maintaining the research's scope and objectives. In future studies focusing on adsorbed layers or more complex interface processes, a different circuit configuration may be more suitable51.
EIS plots of mild steel in 3.5% NaCl solutions without and with different concentrations of polyaniline (a) Nyquist plots (b) Bode plots (c) Phase plots (d) Equivalent circuit.
Deducing whether the capacitance observed in electrochemical impedance spectroscopy (EIS) data corresponds to the double layer or a specific component, such as the capacitance of polyaniline in your case, involves a careful analysis of the frequency response and experimental conditions52. The double-layer capacitance typically manifests as a semicircle in the high-frequency region of the Nyquist plot. The diameter of this semicircle is related to the double-layer capacitance. In the Bode plot, the double-layer capacitance contributes to a phase angle close to -90 degrees at high frequencies. The capacitance associated with the redox processes of polyaniline may be reflected in features like peaks or shoulders in the low-frequency region of the Nyquist plot or deviations from ideal behavior in the Bode plot53. The double-layer capacitance is predominantly observed at higher frequencies, typically above 0.1 Hz. The capacitance associated with the redox processes of polyaniline may dominate at lower frequencies, below 0.1 Hz. The double-layer capacitance is influenced by the nature of the electrolyte and the specific experimental conditions. It is usually more dependent on the electrolyte composition. The capacitance associated with polyaniline may be more influenced by the redox-active properties of the material and the specific electrochemical conditions, such as potential and pH54. Perform EIS measurements at different potentials to explore how the capacitance changes. The double-layer capacitance is potential-dependent, whereas the capacitance associated with polyaniline may exhibit redox peaks or changes in the low-frequency impedance. Conduct control experiments with a system that does not contain polyaniline to observe the baseline behavior of the double-layer capacitance. Use equivalent circuit modeling to fit the EIS data. Include elements representing the double-layer capacitance and other capacitances associated with specific electroactive components like polyaniline. Extract the values of the circuit elements from the fitting, and observe how they correlate with the expected behavior of double-layer capacitance and polyaniline capacitance. Examine previous studies or literature on the EIS of polyaniline to understand the typical frequency response and features associated with polyaniline capacitance. If polyaniline is a significant component in your system, consider collaborating with experts in polyaniline electrochemistry to gain insights into its expected electrochemical behavior in EIS55. By carefully considering these factors and conducting additional experiments, you can improve your confidence in deducing whether the capacitance observed in your EIS data corresponds to the double layer or the capacitance of polyaniline. Keep in mind that the interpretation may be system-specific, and collaboration with experts in relevant fields can be beneficial.
The inherent strength and durability of steel render it a highly sought-after metal for diverse applications. However, the susceptibility of steel to corrosion poses a significant challenge, potentially leading to extensive damage or complete disintegration over time. This vulnerability is particularly pronounced in the case of steel spearheads and other ancient artifacts, emphasizing the critical need for effective corrosion prevention strategies to safeguard these historical objects51. Polyaniline coatings emerge as a novel and promising method for preventing corrosion. Polyaniline, being a conductive polymer, exhibits remarkable barrier properties and the ability to function as a sacrificial anode, providing exceptional protection against corrosion. This study specifically investigates the efficacy of a flawless polyaniline coating in preventing corrosion on steel spearheads. Before assessing the coating's effectiveness, meticulous cleaning and pre-treatment procedures were employed to eliminate any residual corrosion products from the spearheads56. Application of the polyaniline layer was carried out using a straightforward dip-coating method, and the coated spearheads underwent a salt spray test to simulate environmental conditions. The research findings indicate that the polyaniline coating offers superior protection against corrosion. Even after prolonged exposure to the salt spray test, the coated spearheads exhibited minimal deterioration. In stark contrast, untreated spearpoints displayed severe corrosion damage in a relatively short period. The success of the polyaniline coating is attributed to its role as a protective shield, effectively guarding the steel surface against atmospheric corrosion. Additionally, the electrical characteristics of polyaniline make it an ideal sacrificial anode, further enhancing its protective capabilities57. In conclusion, this research underscores the potential of polyaniline coatings as an effective means of safeguarding steel archaeological objects, such as spearheads, against corrosion. The study advocates for further research into the longevity of polyaniline coatings and their applicability to a broader range of steel artifacts58. The mechanisms underlying the corrosion mitigation properties of polyaniline involve the adsorption of corrosive substances present on metal surfaces. Nitrogen and oxygen atoms on the metal surface serve as chelation sites for ions of other elements, facilitating the formation of complexes with organic molecules59. The efficacy of polyaniline in preventing mild steel corrosion in a 3.5% NaCl environment is investigated by examining active sites, the interaction mode with Fe3+ ions, and the formation of stable Fe-polyaniline complexes. The adsorbed layer of polyaniline on the substance's surface plays a crucial role in preventing corrosion, involving both chemisorption and physical adsorption, with electrostatic interactions and charge sharing. The potential existence of Fe-polyaniline complexes on metal surfaces, specifically between steel, oxygen, and nitrogen atoms, contributes to the formation of protective layers. These results align with the hypothesis proposed in the study60,61,62,63.
To ensure the long-term preservation of steel spears obtained from archaeological excavations, conservation treatment becomes imperative. Numerous similar relics are curated at the Military Museum in Al-Qala, Egypt, emphasizing the importance of effective preservation strategies for these historical artifacts. One such approach involves the application of a flawless polyaniline coating, renowned for its conductivity and strong adherence, making it a widely used coating material in conservation efforts64. Polyaniline, owing to its exceptional properties, can withstand environmental challenges, including moisture, sunlight, and temperature fluctuations. The application of a flawless polyaniline coat on steel spears serves as a protective measure against corrosion, acting as a physical barrier between the metal and the external atmosphere, thus preventing deterioration due to rust. Electrochemical polymerization is employed in the process of applying this coating. The procedure initiates with the creation of an aniline monomer solution, through which an electric current is passed, resulting in the formation of a thin polyaniline coating on the steel spear's surface65. Beyond its application on coins and sculptures, polyaniline coatings have proven effective in preserving a variety of ancient tools and metal artifacts. In the context of the Military Museum in Al-Qala, Egypt, the steel spears will benefit from protection against further corrosion through the application of a flawless polyaniline covering66. Conservation practices often involve cleaning, and the choice of a cleaning method depends on factors such as the artifact's composition, current condition, and the desired conservation goal. Given that all the spearpoints exhibit concretion and a hard crust in a similar state, mechanical means of cleaning would be challenging. Hence, a chemical cleaning process assisted by meticulous mechanical cleaning was chosen. The comprehensive therapy included multiple steps, starting with the use of soapy solutions to remove grime and oil. Subsequently, citric acid and thiourea solution were employed to dissolve corrosion, followed by rinsing with deionized water to remove soluble corrosion products. Mechanical brushing and immersion in a sodium hydroxide solution neutralized the acidic state, with subsequent rinsing and drying67. To prevent the accumulation of harmful chemicals between various materials, the spearheads were shielded with a corrosion mitigator. The detailed steps of this procedure are illustrated in Fig. 12a–f. In conclusion, the application of a flawless polyaniline layer proves to be an effective and meticulous strategy for preserving steel spears from archaeological sites. These measures ensure that these invaluable artifacts will endure for future generations to appreciate and study68. The inquiry into the state of decay of a nineteenth century spearhead housed at the Al-Qala Museum in Cairo, Egypt, involved visual inspection, metallographic microscopy, and scanning electron microscopy coupled with energy dispersive spectrometry. Degradation spots, such as pitting surfaces, micro-cracks, and grief corrosion, were identified on the predominantly steel spears. Charcoal was also detected interspersed with steel flakes in the sample. XRD examinations of corrosion products revealed the presence of hematite, ferromagnetite, magnetite, quartz, and calcite. The spearpoints were primarily composed of a low to mid-range carbon percentage alloy of steel. Wrought steel, until the mid-nineteenth century, found applications primarily in tie rods, straps, and nails. Slag, integral to the ductile alpha-steel matrix, plays a role in wrought steel's microstructure. The absence of a passivation layer necessitates corrosion prevention measures for metal items69,70.
(a–e) Show the group of spearheads before treatment and conservation and image (f) represents the group of spearheads after treatment and conservation.
This investigation focuses on a 19th-century spearhead displayed at the Al-Qala Museum in Cairo, Egypt, aiming to assess its state of decay using visual examination as a preliminary method71. To quantify the corrosion rate, estimate the cross-sectional area converted to corrosion products, and determine corrosion depth, analytical techniques such as metallographic microscopy, scanning electron microscopy with energy dispersive spectrometry (SEM&EDS), and an Inspect S 50 (FEI) were employed. The chemical makeup, microstructure, and surface characterization of spear pieces were revealed through these techniques, and the analytical findings are presented in Tables 1 and 2. The examination of corrosion byproducts was conducted using X-ray diffraction (XRD), and Table 3 presents the identified corrosion by-products, recognizing their dependency on the environment72. Metallographic and Scanning Electron Microscopy analyses confirmed the presence of pitting, microcracks, and severe corrosion on the spear tips, as depicted in Figs. 8, 9, 10, 11, 12. The innate inclination of many metals to revert to their more stable, corroded forms underscores the necessity of shielding metal objects from elements and impurities that can induce corrosion. While oxide films developed during corrosion usually act as insulating barriers, providing a protective layer known as a passivation layer, steel seldom forms such layers73. Specific regions shielded by patches of oxide became anodes, while others became cathodes, mimicking miniature galvanic cells in the presence of an electrolyte. Without additional coatings to prevent corrosion, steel will continue to corrode until no metal remains (70). Despite the valuable insights chemical microanalysis can offer for charcoal-reduced steel, metallographic examination revealed details of charcoal between steel grains (Figs. 8, 9, and 10)74. The historical method of recovering steel from ores using charcoal in a small furnace is elucidated, with the resultant "bloom" composed of steel particles and non-metallic ore components forming "slag." The prolonged contact between the bloom and carbon leads to the formation of an alloy of steel and carbon, known as steel. Air cooling results in equilibrium conditions, producing a microscopic structure termed pearlite. Quenching, however, enhances the hardness of steel, with the Vickers pyramid hardness (VPH) scale measuring a metal's hardness. The Carbon/Sulfur Analyzer determined 0.93 wt. % of carbon and 0.15 wt. % of sulfur in the alloy75,76,77,78. The choice of steel alloy for spearheads aligns with historical practices, with cast steel having a high carbon concentration (more than 2% but typically less than 5%) and wrought steel having a low carbon content (not exceeding 0.35%). The predominant applications of wrought steel until the mid-nineteenth century were tie rods, straps, and nails79,80. Wrought steel is characterized by its microstructure, primarily composed of a ductile-steel (ferrite) matrix with slag inclusions81,82,83. The melting point of wrought steel is 1535 °C, and its malleability, ductility, and strength increase with working. X-ray diffraction revealed that hematite, ferroxidase, magnetite, quartz, and calcite are primary components of corrosion products (Fe3O4), with the oxide and hydroxide layers forming on the steel surface providing some protection84,85. The presence of martensite does not necessarily indicate intentional steel use; however, the smith's skill was crucial in producing a uniform metal plate with minimal slag impurities. Corrosion products from the spearheads' metallic parts include Quartz, Calcite, Feroxyhyte, Magnetite, and Hematite. A cleaning procedure involving a 5% Citric Acid solution and 1% Thiourea was employed, followed by mechanical cleaning and a soak in a 2% sodium hydroxide solution. Citric acid, though generally harmless, should be handled cautiously, especially with certain steel types, due to potential hydrogen embrittlement86,87,88,89,90,91,92,93. Mechanical cleaning methods, such as wire brushing, should be performed cautiously, considering the type of steel and the length of acid treatment. Persistent deposits may require additional time for mechanical removal, and citric acid soaks can be employed to complete the cleaning while minimizing further damage. The study underscores the importance of preservation strategies for historical artifacts and provides valuable insights into the composition and corrosion characteristics of the 19th-century steel spearheads94,95,96.
Metallography microscopy, scanning electron microscopy, Energy Dispersive spectroscopy (EDS), X-ray diffraction (XRD), and a carbon/sulfur analyzer were employed to meticulously examine and analyze steel archaeological artifacts. The primary objective was to pinpoint the precise chloride-containing phases responsible for the deterioration of these objects. It was observed that adsorbed chloride (Cl) appears to be present and plays a significant role in the corrosion process, leading to the subsequent deterioration of artifacts during excavation. Factors such as oxygen, water, chloride ions, and airborne contaminants are known contributors to the corrosion of metals. Therefore, it is imperative to establish an environment that offers protection against these corrosive elements. To effectively preserve steel items, they should be exhibited in controlled conditions characterized by stable temperatures, low relative humidity, and an absence of airborne contaminants, including oxygen, water, and chloride ions. It's important to note that plastic bags are not suitable for storing steel items, as they tend to trap moisture, dust, and dirt. This trapped moisture can elevate the relative humidity within the bag, creating a more conducive environment for corrosion. Consequently, it is advisable to use polyethylene bags with strategically placed punctures to allow for air circulation. Avoid the use of polyvinyl chloride (PVC) bags, as they may emit hydrogen chloride, an acidic gas that can corrode most metals. Furthermore, the study's findings indicate that the corrosion of mild steel can be effectively inhibited using a solution containing 3.5% sodium chloride and polyaniline. Notably, the degree of inhibition is directly proportional to the concentration, with inhibition levels reaching approximately 98% at a concentration of 100 ppm. Additionally, the data suggests an inverse relationship between concentration and corrosion inhibitory effect (ppm). Impedance measurements provide further evidence, showing a decrease in the double-layer capacitance of the protective film and an increase in its charge transfer resistance. These results suggest that the protective film is likely to be relatively thick and dense compared to other types of protective films.
Frank, H. Good year, archaeological site science. pp 131–132 (1988).
Yang Sook, K. & Istvan, S. Cleaning of corroded iron artifacts using pulsed TEA CO2 and Nd: YAG lasers. J. Cult. Herit. 4, 129–133 (2003).
Hefter, G., North, A. & Tan, S. Organic corrosion inhibitors in neutral solutions; part-I Inhibition of steel, copper, and aluminum by straight chain carboxylates. Corrosion 53(8), 657–667. https://doi.org/10.5006/1.3290298 (1997).
Alkharafi, F. M., El-Shamy, A. M. & Ateya, B. G. Comparative effect of tolytriazole and benzotriazole against sulfide attack on copper. Int. J. Electrochem. Sci. 4, 1351–1364 (2009).
Mirambet, F., Reguer, S., Rocca, E., Hollner, S. & Testemale, D. A. Complementary set of electrochemical and X-ray synchrotron techniques to determine the passivation mechanism of iron treated in a new corrosion inhibitor solution specifically developed for the preservation of metallic artifacts. Appl. Phys. A 99, 341–349 (2010).
Article ADS CAS Google Scholar
Sherif, E. M., Abbas, A. T., Gopi, D. & El-Shamy, A. M. Corrosion and corrosion inhibition of high strength low alloy steel in 2.0 M sulfuric acid solutions by 3-amino-1,2,3-triazole as a corrosion inhibitor. J. Chem. https://doi.org/10.1155/2014/538794 (2014).
Bethencourt, M., Botana, F. J., Calvino, J. J., Marcos, M. & Rodriguez-Chacon, M. A. Lanthanide compounds as environmentally friendly corrosion inhibitors of aluminum alloys: A review. Corros. Sci. 40(11), 1803–1819. https://doi.org/10.1016/S0010-938X(98)00077-8 (1998).
Sabirneeza, A. A. F., Geethanjali, R. & Subhashini, S. Polymeric corrosion inhibitors for iron and its alloys: A review. Chem. Eng. Commun. 202(22), 232–244. https://doi.org/10.1080/00986445.2014.934448 (2015).
Sherif, E. M., Abbas, A. T., Halfa, H. & El-Shamy, A. M. Corrosion of high strength steel in concentrated sulfuric acid pickling solutions and its inhibition by 3-amino-5-mercapto-1, 2, 3-triazole. Int. J. Electrochem. Sci 10, 1777–1791 (2015).
Dillmann, P., Beranger, G., Piccardo, P. & Matthiessen, H. Corrosion of Metallic Heritage Artefacts: Investigation, Conservation, and Prediction of Long-Term Behavior (Elsevier, 2014).
El-Shamy, A. M., Shehata, M. F. & Ismail, A. I. M. Effect of moisture contents of bentonitic clay on the corrosion behavior of steel pipelines. J. Appl. Clay Sci. 114, 461–466. https://doi.org/10.1016/j.clay.2015.06.041 (2015).
Singh, P., Srivastava, V. & Quraishi, M. A. Novel quinoline derivatives as green corrosion inhibitors for mild steel in acidic medium: electrochemical, SEM, AFM, and XPS studies. J. Mol. Liquids 216(1), 164–173. https://doi.org/10.1016/j.molliq.2015.12.086 (2016).
Farag, HK, El-Shamy, AM, Sherif, EM & El Abedin, SZ Sonochemical Synthesis of Nanostructured ZnO/Ag Composites in an Ionic Liquid.Journal of Physical Chemistry 230(12), 1733–1744.https://doi.org/10.1515/zpch-2016-0777 (2016).
Elsayed, E. M., Eessaa, A. K., Rashad, M. M. & El-Shamy, A. M. Preparation and characterization of ZnO thin film on anodic Al2O3 as a substrate for several applications. Egypt. J. Chem. 65(10), 119–129. https://doi.org/10.21608/ejchem.2022.110382.5021 (2022).
El-Shamy, A. M., Farag, H. K. & Saad, W. M. Comparative study of removal of heavy metals from industrial wastewater using clay and activated carbon in batch and continuous flow systems. Egypt. J. Chem. 60(6), 1165–1175. https://doi.org/10.21608/ejchem.2017.1606.1128 (2017).
Liu, A. M., Ren, X. F., Wang, B., Zhang, J., Yang, P. X., Zhang, J. Q., An, M. Z. Complexing agent study via computational chemistry for environmentally friendly silver (2014).
El-Shamy, A. M., Shehata, M. F., Metwally, H. I. M. & Melegy, A. Corrosion and corrosion inhibition of steel pipelines in montmorilonitic soil filling material. Silicon 10(6), 2809–2815. https://doi.org/10.1007/s12633-018-9821-4 (2017).
Sanatkumar, B., Nayak, J. & Shetty, N. Influence of 2-(4-chlorophenyl)-2-oxoethyl benzoate on the hydrogen evolution and corrosion inhibition of 18 Ni 250 grade weld aged maraging steel in 1.0 M sulfuric acid medium. Int. J. Hydrog. Energy 37(11), 9431–9442. https://doi.org/10.1016/j.ijhydene.2012.02.165 (2012).
Giumlia, M.A., Williams, A. 'In situ' metallographic studies on the armor of the Basilica of the Beata Vergine delle Grazie, Udine, Aquileia Nostra, LXXV Udine, Aquileia, 394–422 (2004).
El-Shamy, A. M. Control of corrosion caused by sulfate-reducing bacteria. In Microbes in process, pp. 337–362 (2014).
Scharff, W. & Huesmann, I. A. Accelerated decay of metal soil finds due to soil pollution. Metal 95, 17–20 (1997).
Ateya, B. G., Al Kharafi, F. M., El-Shamy, A. M., Abdalla, R. M. Electrochemical oxidation of hydrogen sulfide in geothermal fluids under high temperature and pressure. In ACS National Meeting Book of Abstracts 2008 236th National Meeting and Exposition of the American Chemical Society, ACS 200817 August 2008 through 21 August (2008).
Ateya, B. G., Alkharafi, F. M., El-Shamy, A. M., Saad, A. Y. & Abdalla, R. M. Electrochemical desulphurization of geothermal fluids under high temperature and pressure. J. Appl. Electrochem. 39, 383–389. https://doi.org/10.1007/s10800-008-9683-3 (2009).
Abdelshafeek, K. A., Abdallah, W. E., Elsayed, W. M., Eladawy, H. A. & El-Shamy, A. M. Vicia faba peel extracts bearing fatty acids moieties as a cost-effective and green corrosion inhibitor for mild steel in marine water: Computational and electrochemical studies. Sci. Rep. 12(1), 20611. https://doi.org/10.1038/s41598-022-24793-3 (2022).
Article ADS CAS PubMed PubMed Central Google Scholar
Abd Elkarim, A. M., El-Shamy, A. M., Megahed, M. M. & Kalmouch, A. Evaluation of the inhibition efficiency of a new inhibitor on leaded bronze Statues from Yemen. Arctic J. 71(1), 2–33 (2018).
Cano, E. et al. Electrochemical characterization of organic coatings for protection of historic steel artifacts. J. Solid State Electr. 14, 453 (2010).
Where, AK, El-Shamy, AM & Reda, Y. Fabrication of commercial nanoporous alumina by low voltage anodizing.Egypt.J. Chem.61(1), 175–185.https://doi.org/10.21608/ejchem.2017.2189.1175 (2018).
Satri, V. Green Corrosion Inhibitors: Theory and Practice (Wiley, 2011).
El-Shamy, A. M., Abdelfattah, I., Elshafie, O. I. & Shehata, M. F. Potential removal of organic loads from petroleum wastewater and its effect on the corrosion behavior of municipal networks. J. Environ. Manag. 219, 325–331. https://doi.org/10.1016/j.jenvman.2018.04.074 (2018).
Elban, W. L., Borst, M. A., Roubachewsky, N. M., Kemp, E. L. & Tice, P. C. Metallurgical assessment of historic wrought iron: US custom house, wheeling, West Virginia. Assoc. Preserv. Technol. 29, 27–34 (1998).
Bramfitt, B. L., Benscoter, A.O. Metallographer’s guide: Practices and procedures for irons and steels, USA (2002).
Bussell, M. Use of iron and steel in buildings. In Structures & Construction in Historic Building Conservation (ed. Forsyth, M.) 173–191 (Blackwell Publishing Ltd, 2007).
Reda, Y., El-Shamy, AM & Where, AK Effect of hydrogen embrittlement on the microstructures of electroplated steel alloy 4130. Ain Shams Eng.J. 9(4), 2973–2982.https://doi.org/10.1016/j.acej.2018.08.004 (2018).
Al-Otaibi, M. S. et al. Corrosion inhibitory action of some plant extracts on the corrosion of mild steel in acidic media. Arab. J. Chem. 7, 340–346 (2014).
El-Kashef, E., El-Shamy, A. M., Abdo, A., Gad, E. A. M. & Gado, A. A. Effect of magnetic treatment of potable water in looped and dead-end water networks. Egypt. J. Chem. 62(8), 1467–1481. https://doi.org/10.21608/ejchem.2019.7268.1595 (2019).
Zajec, B., Leban, M. B., Lenart, S., Gavin, K. & Legat, A. Electrochemical impedance and electrical resistance sensors for the evaluation of anticorrosive coating degradation. Corros. Rev. 35, 65–74 (2017).
Abbas, M. A., Zakaria, K., El-Shamy, A. M. & El Abedin, S. Z. Utilization of 1-butylpyrrolidinium chloride ionic liquid as an eco-friendly corrosion inhibitor and biocide for oilfield equipment: combined weight loss, electrochemical and SEM studies Z. Phys. Chem. 235(4), 377–406. https://doi.org/10.1515/zpch-2019-1517 (2019).
Xia, D. H. et al. Assessing atmospheric corrosion of metals by a novel electrochemical sensor combining with a thin insulating net using electrochemical noise technique. Sens. Actuat. B Chem. 252, 353–358 (2017).
Shehata, M. F., El-Shafey, S., Ammar, N. A. & El-Shamy, A. M. Reduction of Cu+2 and Ni+2 ions from wastewater using mesoporous adsorbent: Effect of treated wastewater on corrosion behavior of steel pipelines. Egypt. J. Chem. 62(9), 1587–1602. https://doi.org/10.21608/ejchem.2019.7967.1627 (2019).
Scott, D. A. & Eggert, G. Iron and Steel in Art: Corrosion, Colorants, Conservation 145–147 (Archetype Publications, 2009).
El-Shamy, A. M., Soror, T. Y., El-Dahan, H. A., Ghazy, E. A. & Eweas, A. F. Microbial corrosion inhibition of mild steel in salty water environment. Mater. Chem. Phys. 114(1), 156–159. https://doi.org/10.1016/j.matchemphys.2008.09.003 (2009).
Zohdy, K. M., El-Shamy, A. M., Gad, E. A. M. & Kalmouch, A. The corrosion inhibition of (2Z,2′Z)-4,4′-(1,2-phenylene bis (azanediyl)) bis (4-oxobut-2-enoic acid) for carbon steel in acidic media using DFT. Egypt. J. Pet. 28(4), 355–359. https://doi.org/10.1016/j.ejpe.2019.07.001 (2019).
Ma, C. et al. Electrochemical noise monitoring of the atmospheric corrosion of steels: Identifying corrosion form using wavelet analysis. Corros. Eng. Sci. Technol. 52, 432–440 (2017).
Reda, Y., El-Shamy, AM, Zohdy, KM & Where, AK Instrument of chloride ions on the pitting corrosion of electroplated steel alloy 4130. Ain Shams Eng.J. 11, 191–199.https://doi.org/10.1016/j.acej.2019.09.002 (2020).
Cano, E., Lafuente, D. Corrosion inhibitors for the preservation of metallic heritage artifacts. In Corrosion and conservation of cultural heritage metallic artefacts; Elsevier: New York, NY, USA, 570–594 (2013).
Reda, Y., Zohdy, K. M., Eessaa, A. K. & El-Shamy, A. M. Effect of plating materials on the corrosion properties of steel alloy 4130. Egypt. J. Chem. 63(2), 579–597. https://doi.org/10.21608/ejchem.2019.11023.1706 (2020).
Mohamed, W. A. & Mohamed, N. M. Testing coatings for enameled metal artifacts. Int. J. Conserv. Sci. 8, 15–24 (2017).
Mohamed, O. A., Farghali, A. A., Eessaa, A. K. & El-Shamy, A. M. Cost-effective and green additives of pozzolanic material derived from the waste of alum sludge for successful replacement of Portland cement. Sci. Rep. 12(1), 20974. https://doi.org/10.1038/s41598-022-25246-7 (2022).
Article ADS CAS PubMed PubMed Central Google Scholar
Shehata, M. F., El-Shamy, A. M., Zohdy, K. M., Sherif, E. S. M. & El Abedin, S. Z. Studies on the antibacterial influence of two ionic liquids and their corrosion inhibition performance. Appl. Sci. 10(4), 1444. https://doi.org/10.3390/app10041444 (2020).
El-Shamy, A. M., El-Hadek, M. A., Nassef, A. E. & El-Bindary, R. A. Optimization of the influencing variables on the corrosion property of steel alloy 4130 in 3.5 wt.% NaCl solution. J. Chem. https://doi.org/10.1155/2020/9212491 (2020).
Rodgers, B. A. The Archaeologist’s Manual for Conservation 186–200 (Kluwer Academic/Plenum Publishers, 2004).
Devanathan, M. A. V. & Tilak, B. V. K. The structure of the electrical double layer at the metal–solution interface. Chem. Rev. 65, 635–684 (1965).
Parsons, R. Electrical double layer: Recent experimental and theoretical developments. Chem. Rev. 90, 813–826 (1990).
Kolb, D. M., Rath, D. L., Wille, R. & Hansen, W. N. An ESCA study on the electrochemical double layer of emersed electrodes. Ber. Bunsenges. Phys. Chem. 87, 1108–1113 (1983).
Brown, M. A. et al. Determination of surface potential and electrical double-layer structure at the aqueous electrolyte–nanoparticle interface. Phys. Rev. X 6, 011007 (2016).
El-Shamy, A. M., El-Hadek, M. A., Nassef, A. E. & El-Bindary, R. A. Box-Behnken design to enhance the corrosion resistance of high strength steel alloy in 3.5 wt% NaCl solution. Mor. J. Chem. 8(4), 788–800. https://doi.org/10.48317/IMIST.PRSM/morjchem-v8i4.21594 (2020).
Mills, D., Picton, P. & Mularczyk, L. Developments in the electrochemical noise method (ENM) to make it more practical for assessment of anti-corrosive coatings. Electrochim. Acta 124, 199–205 (2014).
El-Shamy, A. M. A review on biocidal activity of some chemical structures and their role in mitigation of microbial corrosion. Egypt. J. Chem. 63(12), 5251–5267. https://doi.org/10.21608/ejchem.2020.32160.2683 (2020).
ASTM b499–09, Standard Test Method for Measurement of Coating Thicknesses by the Magnetic Method: Nonmagnetic Coatings on Magnetic Basis Metals; ASTM International: West Conshohocken, PA, USA (2014).
Megahed, M. M., Youssif, M. & El-Shamy, A. M. Selective formula as a corrosion inhibitor to protect the surfaces of antiquities made of leather-composite brass alloy. Egypt. J. Chem. 63(12), 5269–5287. https://doi.org/10.21608/ejchem.2020.41575.2841 (2020).
ASTM e104–02, Standard Practice for Maintaining Constant Relative Humidity by Means of Aqueous Solutions; ASTM International: West Conshohocken, PA, USA (2012).
Megahed, M. M., Abdel Bar, M. M., Abouelez, E. S. M. & El-Shamy, A. M. Polyamide coating as a potential protective layer against corrosion of iron artifacts. Egypt. J. Chem. 64(10), 5693–5702. https://doi.org/10.21608/ejchem.2021.70550.3555 (2021).
Mabbutt, S., Mills, D. J. & Woodcock, C. P. Developments of the electrochemical noise method (ENM) for more practical assessment of anti-corrosion coatings. Prog. Org. Coat. 59, 192–196 (2007).
Zohdy, K. M., El-Sherif, R. M. & El-Shamy, A. M. Corrosion and passivation behaviors of tin in aqueous solutions of different pH. J. Bio Tribo-Corros. 7(2), 1–7. https://doi.org/10.1007/s40735-021-00515-6 (2021).
Pham, T. D. From fuzzy recurrence plots to scalable recurrence networks of time series. EPL-Europhys. Lett. 118, 20003 (2017).
El-Shamy, A. M. & Abdel Bar, M. M. Ionic liquid as water soluble and potential inhibitor for corrosion and microbial corrosion for iron artifacts. Egypt. J. Chem. 64(4), 1867–1876. https://doi.org/10.21608/ejchem.2021.43786.2887 (2021).
Cazares-Ibanez, E., Vazquez-Coutino, G. A. & Garcia-Ochoa, E. Application of recurrence plots as a new tool in the analysis of electrochemical oscillations of copper. J. Electroanal. Chem. 583, 17–33 (2005).
Zohdy, K. M., El-Sherif, R. M., Ramkumar, S. & El-Shamy, A. M. Quantum and electrochemical studies of the hydrogen evolution findings in corrosion reactions of mild steel in acidic medium. Upstream Oil Gas Technol. 6, 100025. https://doi.org/10.1016/j.upstre.2020.100025 (2021).
Wadsworth, F. B., Heap, J. M. & Dingwell, D. B. Friendly fire: Engineering a fort wall in the iron age. J. Archaeol. Sci. 67, 7–13 (2016).
Gad, E. A. & El-Shamy, A. M. Mechanism of corrosion and microbial corrosion of 1,3-dibutyl thiourea using the quantum chemical calculations. J. Bio Tribo-Corros. 8, 71. https://doi.org/10.1007/s40735-022-00669-x (2022).
Oudbashi, O., Emami, S. M., Ahmadi, H. & Davami, P. Micro-stratigraphical investigation on corrosion layers in ancient bronze artefacts by scanning electron microscopy energy dispersive spectrometry and optical microscopy. Herit. Sci. 1, 21–31 (2013).
Abbas, M. A., Ismail, A. S., Zakaria, K., El-Shamy, A. M. & El Abedin, S. Z. Adsorption, thermodynamic, and quantum chemical investigations of an ionic liquid that inhibits corrosion of carbon steel in chloride solutions. Sci. Rep. 12, 12536. https://doi.org/10.1038/s41598-022-16755-6 (2022).
Article ADS CAS PubMed PubMed Central Google Scholar
Abdel-Karim , AM , El-Shamy , AM & Reda , Y. Corrosion and stress corrosion resistance of Al Zn alloy 7075 by nano-polymeric coatings .J. Bio- Tribe-Corros.8, 57. https://doi.org/10.1007/s40735-022-00656-2 (2022).
El-Shamy, A. M., Cathodic protection in the oil and gas industries. In Corrosion and Materials in the oil and gas industry, pp. 489–510 (2016).
Jegdic, B., Radovanavic, S. P., Ristic, S. & Alil, A. Corrosion Processes nature and composition of corrosion products on iron artefacts of weaponry. Sci. Tech. Rev. 61(2), 50–56 (2011).
Abdel-Karim, A. M. & El-Shamy, A. M. A review on green corrosion inhibitors for protection of archeological metal artifacts. J. Bio- Tribo-Corros. 8, 35. https://doi.org/10.1007/s40735-022-00636-6 (2022).
Webber, C. L. Jr. Recurrence Quantification Analysis: Theory and Best Practices (Springer, 2014).
Landolt, D. Corrosion and Surface Chemistry of Metals, Lausanne, Switzerland (ISBN 978-2-940222-11-7), 119–179 (2007).
Mouneir, S. M., El-Hagrassi, A. M. & El-Shamy, A. M. A review on the chemical compositions of natural products and their role in setting current trends and future goals Egypt. J. Chem. 65(5), 491–506. https://doi.org/10.21608/ejchem.2021.95577.4486 (2022).
Loeper-Attia, M. A. A Proposal to Describe Reactivated Corrosion of Archaeological Iron Objects. In Corrosion of Metallic Heritage Artefacts: Investigation, Conservation, and Prediction For Long-Term Behavior (eds Dillmann, P. et al.) 190–202 (Woodhead Publishing, 2007).
Reda, Y., Yehia, H. M. & El-Shamy, A. M. Microstructural and mechanical properties of Al-Zn alloy 7075 during RRA and triple aging. Egypt. J. Pet. 31, 9–13. https://doi.org/10.1016/j.ejpe.2021.12.001 (2022).
Branzoi, F., Branzoi, V. & Licu, C. Corrosion inhibition of carbon steel in cooling water systems by new organic polymers as green inhibitors. Mater. Corros. Werkstoffe Korrosion 65(6), 637–647. https://doi.org/10.1002/maco.201206579 (2014).
Elsayed, E. M., Eessaa, A. K., Abdelbasir, S. M., Rashad, M. M. & El-Shamy, A. M. El-Fabrication, characterization, and monitoring the propagation of nanocrystalline ZnO thin film on ITO substrate using electrodeposition technique. Egypt. J. Chem. 66(2), 33–43. https://doi.org/10.21608/ejchem.2022.126134.5595 (2023).
El-Shamy, A. M. & Mouneir, S. M. Medicinal materials as eco-friendly corrosion inhibitors for industrial applications: A review. J. Bio Tribo-Corrosion 9(1), 3. https://doi.org/10.1007/s40735-022-00714-9 (2023).
Zohdy, K. M., El-Sherif, R. M. & El-Shamy, A. M. Effect of pH fluctuations on the biodegradability of nanocomposite mg-alloy in simulated bodily fluids. Chem. Paper 77(3), 1317–1337. https://doi.org/10.1007/s11696-022-02544-y (2023).
Alwaleed, R. A., Megahed, M. M., Elamary, R. B., El-Shamy, A. M. & Ali, Y. S. Remediation mechanism of microbial corrosion for iron artifacts buried in soil by using allium sativum (garlic extract) as a natural biocide. Egypt. J. Chem. 66(6), 291–308. https://doi.org/10.21608/ejchem.2022.158454.6850 (2023).
Eessaa, A. K. & El-Shamy, A. M. Review on fabrication, characterization, and applications of porous anodic aluminum oxide films with tunable pore sizes for emerging technologies. Microelectr. Eng. 279, 112061. https://doi.org/10.1016/j.mee.2023.112061 (2023).
Eessaa, A. K., Elkady, O. A. & El-Shamy, A. M. Powder metallurgy as a perfect technique for preparation of Cu-TiO2 composite by identifying their microstructure and optical properties. Sci. Rep. 13(1), 7034. https://doi.org/10.1038/s41598-023-33999-y (2023).
Article ADS CAS PubMed PubMed Central Google Scholar
Ghazy, E. A., Abdel Ghany, N. A. & El-Shamy, A. M. Comparative study of cetyl trimethyl ammonium bromide formaldehyde, and isobutanol against corrosion and microbial corrosion of mild steel in chloride media. J. Bio. Tribo-Corrosion 9, 64. https://doi.org/10.1007/s40735-023-00782-5 (2023).
Abdelshafeek, K. A. & El-Shamy, A. M. Review on glucosinolates: Unveiling their potential applications as drug discovery leads in extraction, isolation, biosynthesis, biological activity, and corrosion protection. Food Biosci. 56, 103071. https://doi.org/10.1016/j.fbio.2023.103071 (2023).
Shehata, M. F. & El-Shamy, A. M. Hydrogen-based failure in oil and gas pipelines a review. Gas Sci. Eng. 115, 204994. https://doi.org/10.1016/j.jgsce.2023.204994 (2023).
Elashery, N. H., Megahed, M. M., El-Shamy, A. M. & Saleh, S. M. Archaeometric characterization and conservation of bronze patina on archaeological axe head in military museum, Cairo. J. Archaeol. Tour. Must 2(1), 23–33 (2023).
Selwyn, L. Metals and Corrosion: A Handbook for Conservation Professional (Canadian Conservation Institute, 2004).
Schaefer, K. & Mills, D. J. The application of organic coatings in the conservation of archaeological objects excavated from the sea. Prog. Org. Coat. 102, 99–106 (2017).
Kiele, E. et al. Methyl-modified hybrid organic-inorganic coatings for the conservation of copper. J. Cult. Herit. 15, 242–249 (2014).
Hollner, S., Mirambet, F., Rocca, E. & Reguer, S. Evaluation of new non-toxic corrosion inhibitors for conservation of iron artifacts. Corros. Eng. Sci. Technol. 45, 362–366 (2010).
Open access funding provided by The Science, Technology & Innovation Funding Authority (STDF) in cooperation with The Egyptian Knowledge Bank (EKB). This work was supported by my own.
Conservation Department, Faculty of Archaeology, Fayoum University, Faiyum, Egypt
Mohamed M. Megahed, Noha H. Elashery & Saleh M. Saleh
Physical Chemistry Department, Electrochemistry, and Corrosion Laboratory, National Research Center, El-Bohouth St. 33, Dokki, P.O. 12622, Giza, Egypt
You can also search for this author in PubMed Google Scholar
You can also search for this author in PubMed Google Scholar
You can also search for this author in PubMed Google Scholar
You can also search for this author in PubMed Google Scholar
M.M.M. and A.M.E.S. analyzed the data and wrote the manuscript. N.H.E.A. and Saleh M. Saleh handling the experimental work and helping in the analysis of data.
Correspondence to Ashraf M. El-Shamy.
The authors declare no competing interests.
Springer Nature remains neutral with regard to jurisdictional claims in published maps and institutional affiliations.
Open Access This article is licensed under a Creative Commons Attribution 4.0 International License, which permits use, sharing, adaptation, distribution and reproduction in any medium or format, as long as you give appropriate credit to the original author(s) and the source, provide a link to the Creative Commons licence, and indicate if changes were made. The images or other third party material in this article are included in the article's Creative Commons licence, unless indicated otherwise in a credit line to the material. If material is not included in the article's Creative Commons licence and your intended use is not permitted by statutory regulation or exceeds the permitted use, you will need to obtain permission directly from the copyright holder. To view a copy of this licence, visit http://creativecommons.org/licenses/by/4.0/.
Megahed, M.M., Elashery, N.H., Saleh, S.M. et al. Flawless polyaniline coating for preservation and corrosion protection of ancient steel spearheads: an archaeological study from military museum, Al-Qala, Egypt. Sci Rep 14, 7215 (2024). https://doi.org/10.1038/s41598-024-57184-x
DOI: https://doi.org/10.1038/s41598-024-57184-x
Anyone you share the following link with will be able to read this content:
Sorry, a shareable link is not currently available for this article.
Provided by the Springer Nature SharedIt content-sharing initiative
By submitting a comment you agree to abide by our Terms and Community Guidelines. If you find something abusive or that does not comply with our terms or guidelines please flag it as inappropriate.
Scientific Reports (Sci Rep) ISSN 2045-2322 (online)
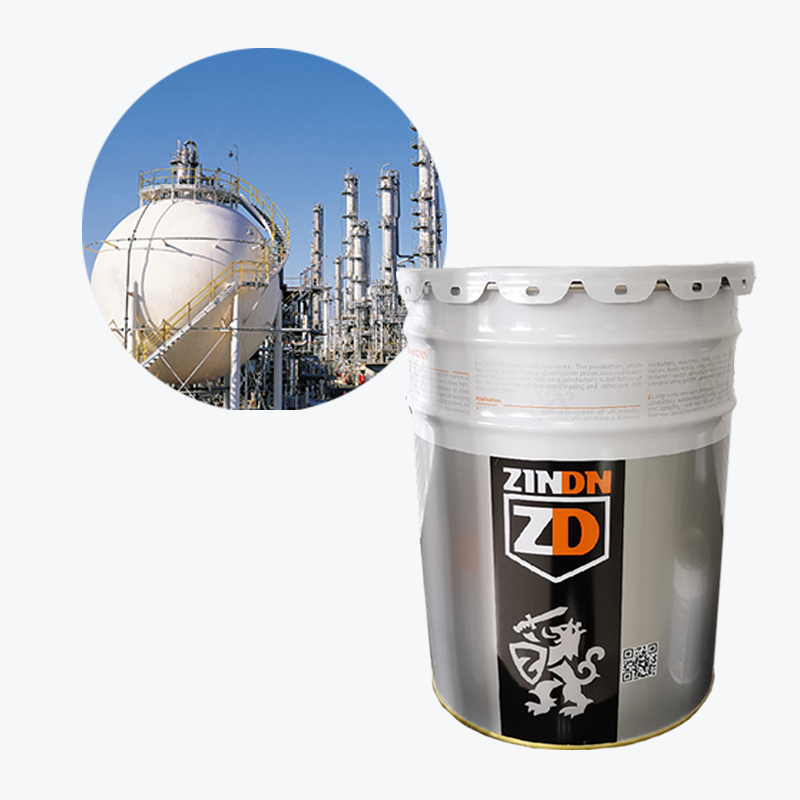
Zinc Rich Primer Sign up for the Nature Briefing newsletter — what matters in science, free to your inbox daily.