Citation numbers are available from Dimensions
China Three Gorges University, China Vinyl Triethoxy Silane
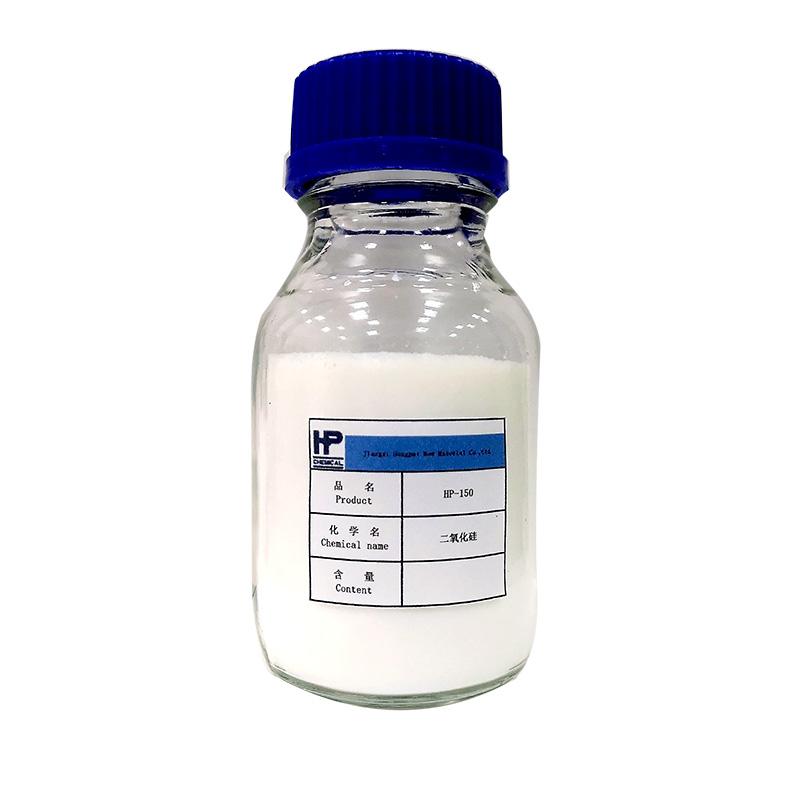
University of Science and Technology Beijing, China
Henan Institute of Engineering, China
Conventional polyvinyl alcohol (PVA) cementitious composites are susceptible to macroscopic cracking when subjected to reduced compressive strength, diminished toughness, tensile impact and other external loads. In order to address these issues, this study investigates the mechanism of the modifier's influence by modifying PVA fibers with a silane coupling agent (KH560) through experimental studies and molecular dynamics simulations for multiscale computational analyses. Macro test results showed that the mechanical properties of PVA fiber concrete were significantly improved after silane coupling agent modified PVA fiber. The microscopic test results showed that the SCA-modified polymer film acted as a bridging ligament in the interfacial transition zone, effectively prevented crack extension, and enhanced the interfacial adhesion between the organic components of the PVA fibers and the cement matrix. Nanoscale molecular dynamics simulations showed that the hydrated calcium silicate exhibited a stable skeletal structure, while the introduction of SCA expanded the hydrogen bonding interactions at the interface, thus enhancing its stability.
Concrete is a crucial construction material in modern engineering, offering a range of advantages including high mechanical strength, good durability, low raw material cost, and easy availability. Cement-based composite materials have emerged as an advancement over traditional cement-based materials. They find extensive application in foundational engineering projects such as buildings, roads, and bridges. However, as material science and construction technology progress, there is a growing demand for improved performance from building materials and structures. Cement-based composites, despite their strengths, suffer from drawbacks like low tensile strength and poor toughness. They tend to develop macroscopic cracks under loads such as tension, impact, and bending. These cracks continue to propagate, eventually leading to brittle fracture and significantly reducing the service life of the composite (Sagar and PrasadKumar, 2012; Mayercsik et al., 2015). Extensive research has demonstrated that the incorporation of fibers in cement-based composites can address these issues by bridging cracks and enhancing load transmission. This, in turn, significantly improves the mechanical properties, durability, and toughness of the composites (Dong and HuangShu, 2013; Yong and DajingChen, 2023). Currently, commonly used fibers in cement-based composites include polyvinyl alcohol fibers, polypropylene fibers, and steel fibers (Zhou et al., 2018; Zhuang et al., 2020; Mei-Ling et al., 2022; LamenJohn, 2023). Polyvinyl alcohol fiber (Said et al., 2021), also known as PVA (Wang and YangYin, 2021), is a novel synthetic fiber with a molecular formula of -[CH2CH(OH)]n- (Guoliang et al., 2023). PVA possesses a unique molecular structure, rendering it a high-elasticity, high-modulus fiber and an environmentally friendly reinforcement material for cement. Its molecular structure facilitates better affinity and interfacial bonding with the cement matrix, thereby expanding the bond-forming surface (Yong and DajingChen, 2023). Moreover, PVA exhibits strong resistance to acids and alkalis, offers a wide range of applications, demonstrates good dispersion performance, and can establish a stable spatial network structure within the concrete system. Several studies have contributed to our understanding of PVA fiber reinforced concrete. Wang et al. (2018) conducted compression tests on PVA fiber rubber concrete and observed a significant reduction in the modulus of elasticity compared to conventional concrete due to the absence of coarse aggregates. Qiu and LimYang (2016) investigated the degradation of the PVA microfiber and the interface with the cement matrix under fatigue loads through single fiber fatigue pulling tests. The study shed light on the mechanisms involved in the degradation of the fiber-matrix interface microstructure. BaominYunqing (2021) examined the effects of partially hydrolyzed and fully hydrolyzed PVA on the physical and bonding properties of fresh and hardened cement mortar. The findings indicated that both partially hydrolyzed and fully hydrolyzed PVA can considerably enhance the bonding properties of cement mortar. In summary, research on polyvinyl alcohol fiber reinforced concrete has yielded significant advancements in building materials and structures (Lisheng and YaxingShunlei, 2022; Wenping et al., 2022).
However, it is important to note that the addition of PVA to concrete can lead to a slight reduction in compressive strength. Therefore, an alternative approach to address this issue is the utilization of modifiers. The modification of concrete through the use of silane coupling agents has gained significant development. Due to the impact of their unique molecular structure on the performance of cement-based materials, researchers have explored the application of silane coupling agents in cement-based materials. In cement paste, the hydroxyl group (-OH) present on the surface of cement particles and their hydration products can undergo condensation with the silicon hydroxyl group (≡Si-OH) in the hydrolyzate of the silane coupling agent, resulting in the formation of a covalent bond (Liu et al., 2020). Franceschini et al. (2007) conducted an analysis that confirmed the chemical bonding between the silane hydroxyl groups in the hydrolyzed product of the silane coupling agent and the surface of the C-S-H gel. This indicates that the silane coupling agent can serve as a connector between the organic polymer and the hydration product. Similarly, Minet et al. (2006) utilized this technique to demonstrate that the silane coupling agent can establish a connection with the C-S-H crystal without damaging the inorganic framework of the C-S-H gel. In another study by Dong and HuangShu (2013) a silane coupling agent was applied to the surface of rubber particles, which were then incorporated into concrete. Through the bridging effect of the silane coupling agent between the rubber particles and the cement matrix, the interface bond strength between the cement matrix and rubber was enhanced. As a result, the mechanical properties of the composite concrete, including improvements of 10%–20%, were observed. These findings highlight the potential of using silane coupling agents as a means to improve the performance of cement-based materials. By establishing stronger interface bonds, these agents offer a promising approach to enhance the mechanical properties of composite concrete while minimizing the reduction in compressive strength. Further research in this area is warranted to explore the full potential and optimize the application of silane coupling agents in cement-based materials.
The raw materials used in this experiment are PVA fiber, stone, sand, cement, and KH-560 coupling agent, whose main materials are shown in Figure 1.
FIGURE 1. Main materials for the experiment. (A) Sand (B) Stones (C) PVA fiber (D) KH560.
The sand and stone used in this experiment are mainly provided by the laboratory of Henan University of Technology, in which the fineness modulus of sand is 2.5 and the particle grading of stone is 5–25 mm; the cement is provided by Zoomlion Cement Xingtai Co., Ltd. and P-O42.5 ordinary silicate cement is selected, and the main chemical compositions are shown in Table 1; the PVA fibers are provided by Changzhou Tianyi Engineering Fibers Co., Ltd. and the physical properties are shown in Table 2; KH-560 coupling agent is provided by Jinan Xingfeilong Chemical Co., Ltd., the physical properties are shown in Table 3; fine aggregate is made of river sand with fineness modulus of 2.5, apparent density of 2,650 kg/m³, bulk density of 1,850 kg/m³; coarse aggregate is continuously graded (5–25 mm) gravel with bulk density of 1,550 kg/m³, apparent density of 2,703 kg/m³.
TABLE 1. Physical and mechanical properties of cement.
TABLE 2. Physical and mechanical properties of PVA fiber.
TABLE 3. Technical indexes of silane coupling agent-KH560.
As shown in Figure 2, the PVA fibers were soaked in anhydrous ethanol for 12 h to remove residues, washed, and dried to constant weight. The deionized water and ethanol solution was stirred well in a volume ratio of 95:5, and acetic acid was added slowly to bring the pH of the solution to about 4.0, and a certain mass of SCA was added after this. The coupling agent prepared as described above was then slowly poured into a container containing the PVA fibers and stirred for 5 min in order to make a homogeneous mixture. It was left at 20°C for 6 h, filtered and then cooled to room temperature, then dried at 40°C to constant weight and set aside.
FIGURE 2. PVA fiber treatment process.
In this paper, the coupling agent KH560 is used as a modifier for PVA fibers. KH560 (C9H20O5Si) has a reactive epoxy group in its molecular structure, which allows it to react with various compounds containing reactive epoxy groups by addition or hydrogen bonding, and to bond firmly with organic polymers (Li et al., 2016a). Notably, KH560 is hydrolyzable in a mixture of ethanol and water. The hydrolysis process is mainly due to the fact that the methoxy (-OCH3) in SCA is a hydrolyzable group, which hydrolyzes in water to form Si-OH hydroxyl groups and -OH bonds. In addition, if the dosage is high, a -OH bond self-condensation reaction may occur, and the Si-O-Si bond formed can react with inorganic materials containing hydroxyl groups (Bingxiang et al., 2022a). Many -OH bonds are present on the surface of PVA fibers treated with coupling agent KH560, and -OH bonds and Ca(OH)2 products are present in the cement hydration products. This makes it easy to form chemical bond connections at the interface between organic materials and cement hydration products. In other words, the Si-OH groups in the coupling agent KH560 and the -OH groups in the hydration products can be condensed and merged to form Si-O-Ca bonds, which can increase the van der Waals interaction force at the interface, and the hydrogen bonding between the hydroxyl groups can further improve the interfacial adhesion.
In order to understand the effect of SCA on the mechanical properties of PVA fibre concrete, mechanical tests were carried out on composite concrete samples that reached the curing target period (7–28 days). In this study, the relevant mechanical property tests were conducted in accordance with the standard “Test Methods for Physical and Mechanical Properties of Concrete” (GB/T50008-2019). As shown in Figures 3A, B, the size of the specimen block used for the cube compressive strength test was 100 mm × 100 mm × 100 mm, and the size of the bending strength specimen was 40 mm × 40 mm × 160 mm. In addition, the Y250 digital electric stress type straight shear and DKZ-5000 electric bending tester were used for the compressive strength and bending strength tests, respectively.
FIGURE 3. The schematic illustration of (A) pressure and (B) flexural test of samples.
Firstly, the specimens cured to the specified age were cut into 10 mm × 10 mm × 10 mm cubes, polished with 2000 grit sandpaper and the hydration reaction was terminated by immersion in anhydrous ethanol. Then, they were placed in a vacuum drying oven at 40°C for 24 h and the samples were filtered by blowing air, and finally the composite interfaces were fixed on a sample stage for vacuum gold plating. Then, Quanta FEG series scanning electron microscope equipment was used to observe the microscopic morphology of the interface with the cement matrix before and after SCA-modified PVA fibers. The schematic diagrams of the compressive (a) and flexural (b) tests of the samples are shown in Figure 3.
In order to further investigate the mechanism of synergistic enhancement of PVA fibre composite cementitious by SCA coupling agent on molecular scale, molecular dynamics simulation of composite cementitious is required. In this paper, molecular dynamics simulations of the interfacial model of PVA cementitious with and without SCA modification were carried out on the nanoscale, respectively, by using Materials Studio (MS) software (Feng et al., 2021; Feng and QinZhao, 2023). MS software can build a set of effective models and algorithms at the atomic level, and apply the classical mechanics method to study the laws of motion of microscopic molecules in order to obtain the basic laws and macroscopic properties of the system. And on this basis, macro and microscopic tests are combined to complete further research on new composite materials (Yang and Cao, 2021).
The performance of concrete is primarily influenced by the hydration products of cement, with the key component being calcium silicate hydrate gel (C-S-H). The C-S-H gel, which is an incomplete crystal, has been extensively studied regarding its structure and dynamics (Tu et al., 2022). Among various models, the Tobermorite molecular structure has proven successful in characterizing the properties of C-S-H during its evolution. Therefore, this study adopts the Tobermorite model as the basis for this investigation (BowersKirkpatrick, 2009; Churakov, 2009). Initially, the Tobermorite crystal is cut, expanded, and combined into a plane along the crystal plane (0 0 1) with parameters U = 22.00 Å and V = 22.00 Å. This plane is then used to construct gel pores, serving as the cement-based model. The chosen PVA type in this study has a density of 1.0 kg/m3. The amorphous unit of the PVA polymer chain consists of three PVA polymer chains, with each chain comprising 30 PVA monomers. The amorphous unit has dimensions of a = 20.20 Å, b = 20.20 Å, c = 20.87 Å, and α = β = γ = 90°. KH560, an amorphous battery composed of 10 molecules with a density of 1.047 kg/m3, has dimensions of a = 22.00 Å, b = 22.00 Å, c = 6.33 Å, and α = β = γ = 90°. Using the Build Layer module, the PVA/C-S-H model (a = 21.40 Å, b = 21.40 Å, c = 46.61 Å) and the PVA/SCA/C-S-H model (a = 21.80 Å, b = 21.80 Å, c = 77.68 Å) were constructed. It should be noted that in these models, the PVA and SCA components do not have fixed surfaces. This is because they can be energetically optimized to assume more suitable positions. Figure 4 (referenced below) provides a visual representation of the construction process for the PVA/C-S-H model (Note: Figure 4 is not provided in the text. Please refer to the original source for the specific figure).
FIGURE 4. PVA/C-S-H Model building process.
In this study, Materials Studio 2020 software was employed for simulating and analyzing all models. The selection of a suitable force field is crucial in molecular dynamics simulations, as it directly impacts the accuracy of the calculation results. After consulting relevant research findings, the Compass (Condensed-phase Optimized Molecular Potential for Atomistic Simulation Studies) force field was chosen for this investigation (Al-Ostaz et al., 2010; Feng et al., 2023). The Compass force field provides a reasonable model for describing organic and inorganic systems, and it has been extensively validated by concrete research scholars, demonstrating high compatibility with cement-based materials and reducing potential errors in the test data. To ensure the convergence of the models and eliminate any unreasonable structural components introduced during the model construction process, the geometry of the two interface models was initially optimized (Bai et al., 2023). Subsequently, an isothermal and isobaric (NPT) equilibrium run was conducted using a time step of 300 ps and 1 fs, with the simulation temperature set to 300 K. The system temperature and pressure were controlled using the Andersen and Berendsen methods, respectively. To strike a balance between simulation accuracy and duration, a dynamic simulation was performed under the NVT ensemble (Bingxiang et al., 2022b). This simulation utilized a time step of 1 fs and a total simulation time of 1 ns, resulting in the generation of the final simulation file. By following these procedures, the study aimed to obtain reliable simulation results while considering the trade-off between accuracy and computational efficiency.
Figure 5 shows the test results of compressive properties of PVA concrete with and without added silane coupling agent at 7 and 28 days of curing, while the results of mechanical properties of plain concrete were used as a control group. The test results show that the compressive and flexural strengths of the concrete were increased to different degrees after incorporation of SCA. Compared with the results of the blank control group, the compressive strength of concrete increased by 0.70 and 0.37 times at 7 and 28 days, respectively, after the incorporation of PVA fibers, which is due to the fact that the incorporation of PVA fibers caused changes in the internal structure of the self-compacting concrete body, which led to an increase in the number of interfaces between the self-compacting concrete and the cementitious matrix, and an increase in the number of internal weak surfaces in the body, which in turn lowered the compressive strength of the PVA concrete. In addition, the compressive strength of PVA/SCA concrete was increased more significantly, by 1.58 and 0.62 times at 7 and 28 days, respectively. It can be seen that PVA fibers modified by SCA can effectively improve the compressive properties of PVA concrete, and the improvement effect is more and more obvious with the increase of curing time.
FIGURE 5. Compressive strength test results of mechanical properties.
Figure 6 shows the test results of the flexural strength of the three types of concrete at curing times of 7 and 28 days. The flexural strength of PVA concrete at 7 and 28 days increased by 0.72 and 0.35 times, respectively, and the compressive strength of PVA/SCA concrete increased by 1.68 and 1.01 times, respectively, compared to the control. The results showed that the flexural properties of PVA concrete with SCA were significantly improved compared to PVA concrete without modifier. It is proved that PVA fibers can reduce the brittle damage of concrete and improve its toughness. In addition, the bridging effect of SCA can improve the weak interfacial transition zone between the polymer and the cement matrix and improve the mechanical properties of the composite concrete. This hypothesis will be verified by SEM and molecular dynamics simulations in the following sections.
FIGURE 6. Flexural strength test results of mechanical properties.
As shown in Figures 7A, B, the smooth traces left by the shedding of PVA fibers and the presence of a large gap at the interface between the cementitious and PVA fibers can be seen, indicating that the unmodified PVA fibers are not tightly bonded to the cementitious, which may lead to cracking of the composite concrete when it is subjected to external loading, and thus indirectly lead to the degradation of its mechanical properties. It is worth noting that the treated PVA fibers were embedded in the concrete without obvious interfacial gaps. This is due to the coupling effect between SCA and the interfacial materials after the PVA fibers are treated with SCA, and this interaction optimizes the hydrophilic fibers of PVA so that they can be more easily bonded with the gel material, thus leading to a significant improvement in their adhesion to the cement matrix. It can be seen that the SCA-modified polymer membrane can act as a bridging ligament at the interface, inhibit the extension of cracks, enhance the interfacial bond between the PVA fiber organic material and the gel material, and play an important role in improving the mechanical properties of PVA composite concrete.
FIGURE 7. The SEM photos of the interface between PVA/cement paste before and after modification. (A) KH560 unmodified PVA fiber PVC/grout interface. (B) KH560 modified PVA fiber PVC/grout interface.
Concentration distribution curves are used to reflect the spatial location of key molecules in the interfacial model and to characterize the molecular state, which is able to reflect the local structure of the modifier and the cement material (Li et al., 2016b; Quan et al., 2021). Due to the limitations of the modeling direction and cycle boundaries, it is necessary to define the bottom of the model as the starting point (0 distance) and analyze the distribution of the concentration function only in the Z direction. In the calculation process, the region along the Z-direction of the model is divided into several cells, the relevant atoms are counted, and the relative concentration can be expressed by Eq. 1:
Figure 8 represents the atomic concentration distribution function of major elements along the Z direction before and after coupling agent addition. As can be seen from the figure, the atomic concentration distribution is nearly symmetric and perpendicular to the nanopore substrate. Within the C-S-H region, Si and Hcw show multiple strong peaks, which indicates that the nanopore has a high affinity for water molecules in a layered structure. In Figure 9A, the atomic concentrations of Sic, Cpva, and Hpva in the 11–16 and 30–33 Å regions show linear fluctuations, suggesting that the number of molecules in this range is low and that nanogaps and simulated boundary effects may exist. In Figure 9B, it is noteworthy that Cpva and Hpva show peaks on both sides of the nanopore and the atomic density distribution within the SCA system in the 20–30 and 45–60 Å regions appears to be disordered, which suggests that there is an interaction between the PVA and coupling agent atoms and that the two have a good coordination ratio. In other words, the incorporation of SCA makes it possible to have a favorable arrangement and bonding between the atoms at the interface between PVA and cement, which greatly improves the interfacial bond strength between the composites.
FIGURE8. Relative concentration distribution of (A) PVA/CSH; and (B) PVA/SCA/CSH along the Z-direction of the system. Si and Hcw, silicic and hydrogen atom where subscript wrepresents water molecule; Cpva and Hpva, carbon and hydrogen atom in PVA molecule respectively; and Csca, Osca, and Hsca = carbon, oxygen, and hydrogen atoms in SCA molecule, respectively.
FIGURE9. MSD evolution of Ca, O, C, and H atoms from (A) PVA–C-S-H; (B) PVA–SCA–C-S-H; and (C) PVA–SCA–C-S-H system at 1,000 ps.
The root mean square displacement (MSD) serves as a significant parameter for evaluating the kinematic properties of atoms. It is calculated as the average of the squared particle displacements. The slope of the MSD curve determines the molecular diffusion coefficient, which increases with the curve’s steepness. It is, therefore, a valuable tool for assessing atomic motion (XuWang, 2018; Feng et al., 2022). The root mean square displacement and time-dependent function can not only provide a visualization of the stability of the interface between PVA and cement, but also allow for characterization of kinetic behaviour prior to and post SCA treatment. To determine the root mean square displacement of the model, initially, we measure the deviation of the atoms from their starting positions over time as a quantifiable displacement. This approach enables us to compare the atomic variances and intermolecular forces in diverse systems (Liu et al., 2021),. The formula used for calculation is listed below.
rit is the position vector of particle I at time t, ri0 is the position vector of particle I at the initial time, represents the average of all atoms in the group. The larger MSD value at time t indicates that the atom diffuses rapidly and moves farther away from its original position.
Figure 9 shows the MSD values of atoms in PVA-C-S-H and PVA/SCA/C-S-H and PVA/SCA/C-S-H, respectively, for discussing the effect of coupling agent on the dynamic properties of PVA fiber concrete. It can be found that the MSD values of H atoms in PVA-C-S-H are higher than the other atoms, on the contrary, the MSD values of Ca and Ocsh atoms in C-S-H are smaller, which indicates that the reactive H atoms at the interface are unstable and have a high degree of activity, whereas the hydrated calcium silicate is more stable after the chemical reaction occurs, and it also indicates that it has a stable skeleton structure. In addition, the MSD values of Figure 9B are all lower than that of Figure 9A, especially the Hpva curve has the largest decrease in MSD value, which is due to the fact that the incorporation of SCA not only reduces the free stretching motions of the Hpva, Ca, and Ocsh atoms at the interface but also increases the hydrogen bonding interactions on the interfaces (Hsca- Hw, Hpva- Ow, and Hsca-Hpva), thus improves the stability of the interface. It is noteworthy that the MSD values of the major atoms decreased before and after SCA addition, but their order did not change. This indicates that the introduction of the coupling agent does not affect the stability of the two systems, but only plays an inhibitory role to enhance the bonding of the PVA-cementitious interface.
In Materials Studio 2020, the binding energy is used to evaluate the interaction strength between different components in the interface model, which represents the energy difference between the total energy of the system and the separation energy of the two parts. It is not only an important parameter reflecting the interfacial interaction, but also a key indicator to measure the adsorption strength during the adsorption process (Caihua et al., 2021; Yong et al., 2021). PVA/CSH binding energy can be calculated by formula (3), and PVA/SCA/CSH formula (4).
The results of the analysis can be used to assess the stability, strength and kinetic properties of the main atoms of the interfacial model under the conditions of the presence or absence of coupling agent. If the result of the binding energy calculation is positive, the two systems are attracted to each other; if the result of the binding energy is negative, these two systems maintain each other; the larger the absolute value of the binding energy, the stronger the interaction between the two systems and the more stable the system is. From Figure 10, it can be seen that the introduction of coupling agent makes the binding energies of both SCA and C-S-H significantly improved, which is due to the hydrophobicity of PVA molecules, and the chemical bond formed between the H atoms of SCA and the H atoms of the PVA fibers by the addition of SCA can improve this hydrophobicity. At the same time, it is considered that the interaction energy is provided by non-bonding interactions, which mainly consist of van der Waals and electrostatic interaction forces between atoms. The absolute value of the energy of the binding energy is significantly larger after the modification of SCA than before the modification, which mainly relies on the hydrogen bonding formed by the H and O atoms of the coupling agent with the water molecules in the PVA and the hydrated calcium silicate, as well as the ionic bonding with Ca, which is important for the enhancement of the PVA cementitious interface.
FIGURE 10. Interaction of PVA/C-S-H, and PVA/SCA/C-S-H interfaces.
In Figure 11, it is shown that when composite concrete is subjected to compression, shear, or bending forces, there is a chemical bond formed between the mortar and the aggregate, as well as between the mortar and the coarse aggregate. Additionally, PVA fibers exhibit good tensile properties, and their addition significantly enhances the shear and flexural properties of the test blocks (Bai et al., 2019). However, it is observed that as the amount of PVA fiber increases, the mechanical properties of PVA concrete start to decrease or even deform due to the relatively weak adhesion between PVA and mortar. In order to address this issue, KH560 is introduced as a reinforcing agent to enhance the interfacial bonding between PVA and cement. The addition of KH560 ultimately improves the mechanical properties of PVA concrete specimens and ensures the specimens remain in good compactness.
FIGURE11. Failure mechanism diagram of different macroscopic test blocks.
In this study, the synergistic strengthening effect of coupling agent-modified PVA fibers on cementitious interfaces at different scales was investigated by combining macroscopic tests, molecular dynamics simulation and analysis. First, at the macro-scale, the effects of different SCA (KH560) contents on the mechanical properties of modified PVA composite concrete were investigated and compared by macroscopic mechanical property tests. At the micro-scale, the effect of SCA on the microstructure of the PVA cementitious interface was observed and analyzed by scanning electron microscopy. On the nanoscale, the molecular dynamics simulation and analysis of the two interfacial models with and without SCA were carried out respectively, and the synergistic reinforcement of the interface was investigated by analyzing the concentration of the main atoms, the root-mean-square displacements and the binding energy. Based on the above comprehensive analysis and study, the following conclusions were drawn:
(1) Macro-mechanical test results show that the addition of SCA can improve the mechanical properties of PVA composite concrete, and PVA fibers can reduce the brittle damage of concrete and improve its toughness.
(2) SEM observation shows that there are obvious cracks at the interface between PVA and cementitious before modification, while the cracks between the two phases are not obvious after modification, but are filled instead, which effectively modifies the interfacial defects between PVA and cementitious, and strengthens the interfacial bonding ability between PVA fiber organic materials and cementitious materials.
(3) In the modeling and analysis of molecular dynamics, we found that the hydrated calcium silicate has a stable skeleton structure, and the addition of SCA increases the hydrogen bonding interactions at the interface, making the PVA-cement interface more compact and stable.
The raw data supporting the conclusion of this article will be made available by the authors, without undue reservation.
CZ: Writing–original draft. LL: Writing–review and editing. XL: Writing–original draft, Writing–review and editing. WC: Writing–review and editing. YL: Writing–review and editing.
The author(s) declare financial support was received for the research, authorship, and/or publication of this article. This research was funded by the 2023 Henan Province Science and Technology Research Project (232102320173, 232102111126). This research was funded by the 2021 Henan Higher Education Teaching Reform Research and Practice Project (2021SJGLX670). This research was funded by the Project type: 2022 Special project of Science and Technology Development Center of Ministry of Education (Grant No. ZJXF2022254).
The authors declare that the research was conducted in the absence of any commercial or financial relationships that could be construed as a potential conflict of interest.
All claims expressed in this article are solely those of the authors and do not necessarily represent those of their affiliated organizations, or those of the publisher, the editors and the reviewers. Any product that may be evaluated in this article, or claim that may be made by its manufacturer, is not guaranteed or endorsed by the publisher.
Al-Ostaz, A., Wu, W., Cheng, A. H. D., and Song, C. (2010). A molecular dynamics and microporomechanics study on the mechanical properties of major constituents of hydrated cement. Compos. PART B-ENGINEERING 41 (7), 543–549. doi:10.1016/j.compositesb.2010.06.005
CrossRef Full Text | Google Scholar
Bai, B., Bai, F., Nie, Q., et al. (2023). A high-strength red mud-fly ash geopolymer and the implications of curing temperature. Powder Technol., 416. doi:10.1016/J.POWTEC.2023.118242
CrossRef Full Text | Google Scholar
Bai, B., Rao, D., Chang, T., and Guo, Z. (2019). A nonlinear attachment-detachment model with adsorption hysteresis for suspension-colloidal transport in porous media. J. Hydrology 578, 124080. doi:10.1016/j.jhydrol.2019.124080
CrossRef Full Text | Google Scholar
BaominYunqing, W. X. (2021). Effect of degree of hydrolysis of polyvinyl alcohol on adhesive properties of cement mortar. J. Test. Eval. 49 (4), 20190036. doi:10.1520/jte20190036
CrossRef Full Text | Google Scholar
Bingxiang, Y., Weijie, C., Jin, Z., Liu, F., Guo, Y., et al. (2022a). Addition of alkaline solutions and fibers for the reinforcement of kaolinite-containing granite residual soil. Appl. Clay Sci. 228, 106644. doi:10.1016/j.clay.2022.106644
CrossRef Full Text | Google Scholar
Bingxiang, Y., Weijie, C., Zihao, L., Zhao, J., Luo, Q., Chen, W., et al. (2022b). Sustainability of the polymer SH reinforced recycled granite residual soil: properties, physicochemical mechanism, and applications. J. Soils Sediments 23 (1), 246–262. doi:10.1007/s11368-022-03294-w
CrossRef Full Text | Google Scholar
BowersKirkpatrick, G. M. R. J. (2009). Natural Abundance43Ca NMR spectroscopy of tobermorite and jennite: model compounds for C-S-H. J. Am. Ceram. Soc. 92 (2), 545–548. doi:10.1111/j.1551-2916.2008.02906.x
CrossRef Full Text | Google Scholar
Caihua, Y., Kui, H., Qilin, Y., Wang, D., Zhang, W., Chen, G., et al. (2021). Analysis of the storage stability property of carbon nanotube/recycled polyethylene-modified asphalt using molecular dynamics simulations. Polymers 13 (10), 1658. doi:10.3390/polym13101658
PubMed Abstract | CrossRef Full Text | Google Scholar
Churakov, S. V. (2009). Structure of the interlayer in normal 11 A tobermorite from an ab initio study. Eur. J. MINERALOGY 21 (1), 261–271. doi:10.1127/0935-1221/2009/0021-1865
CrossRef Full Text | Google Scholar
Dong, Q., and HuangShu, B. X. (2013). Rubber modified concrete improved by chemically active coating and silane coupling agent. Constr. Build. Mater. 48, 116–123. doi:10.1016/j.conbuildmat.2013.06.072
CrossRef Full Text | Google Scholar
Feng, Y., Li, Y., Zhao, C., Dajing, Q., Chao, W., and PeiYan, W. (2022). Nano-CaCO3 enhances PVA fiber-matrix interfacial properties: an experimental and molecular dynamics study. Mol. Simul. 48 (15), 1378–1392. doi:10.1080/08927022.2022.2094373
CrossRef Full Text | Google Scholar
Feng, Y., Qin, D., Li, L., Li, Y., Wang, C., and Wang, P. (2021). EVA enhances the interfacial strength of EPS concrete: a molecular dynamics study. J. Exp. Nanosci. 16 (1), 382–396. doi:10.1080/17458080.2021.2003338
CrossRef Full Text | Google Scholar
Feng, Y., and QinZhao, D. C. (2023). The synergistic strengthening effect of silane coupling agent on the interface between PVA/EPS and cement: experiment and molecular simulation. Compos. INTERFACES 30 (1), 21–41. doi:10.1080/09276440.2022.2109788
CrossRef Full Text | Google Scholar
Feng, Y., Wang, W., Wang, S., Niu, Z., and Li, L. (2023). Multi-scale analysis of mechanical properties of KH-560 coupling agent modified PVA fiber-rubber concrete. Compos. INTERFACES 30 (9), 983–1010. doi:10.1080/09276440.2023.2179267
CrossRef Full Text | Google Scholar
Franceschini, A., AbramsonMancini, S. V., Bresson, B., Chassenieux, C., and Lequeux, N. (2007). New covalent bonded polymer-calcium silicate hydrate composites. J. Mater. Chem. An Interdiscip. J. Deal. Synthesis 17 (9), 913–922. doi:10.1039/b613077a
CrossRef Full Text | Google Scholar
Guoliang, Y., Zhiwen, D., Jingjiu, B., et al. (2023). Experimental study on the dynamic splitting tensile properties of polyvinyl-alcohol-fiber-reinforced cementitious composites. Constr. Build. Mater., 383. doi:10.1016/J.CONBUILDMAT.2023.131233
CrossRef Full Text | Google Scholar
LamenJohn, S. F. (2023). Long-term loss of tension stiffening of concrete containing recycled aggregate and steel fibres. Structures 54, 1312–1319. doi:10.1016/j.istruc.2023.05.145
CrossRef Full Text | Google Scholar
Li, G., Wang, Z., Leung, C. K. Y., Tang, S., Pan, J., Huang, W., et al. (2016a). Properties of rubberized concrete modified by using silane coupling agent and carboxylated SBR. J. Clean. Prod. 112, 797–807. doi:10.1016/j.jclepro.2015.06.099
CrossRef Full Text | Google Scholar
Li, G., Wang, Z., Leung, C. K. Y., Tang, S., Pan, J., Huang, W., et al. (2016b). Properties of rubberized concrete modified by using silane coupling agent and carboxylated SBR. J. Clean. Prod. 112, 797–807. doi:10.1016/j.jclepro.2015.06.099
CrossRef Full Text | Google Scholar
Lisheng, L., and YaxingShunlei, X. H. (2022). Bending and crack evolution behaviors of cemented soil reinforced with surface modified PVA fiber. Materials 15 (14), 4799. doi:10.3390/ma15144799
PubMed Abstract | CrossRef Full Text | Google Scholar
Liu, Q., Wu, J., Xie, L., et al. (2021). Micro-scale investigation of aging gradient within bitumen film around air-binder interface. Fuel, 286. doi:10.1016/j.fuel.2020.119404
CrossRef Full Text | Google Scholar
Liu, T., Wei, H., Zhou, A., et al. (2020). Multiscale investigation on tensile properties of ultra-high performance concrete with silane coupling agent modified steel fibers. Cem. Concr. Compos., 111. doi:10.1016/j.cemconcomp.2020.103638
CrossRef Full Text | Google Scholar
Mayercsik, N. P., Shaeffer, M., Graham-Brady, L., and Kurtis, K. E. (2015). Analysis of Portland cement mortar under impact: a combined material characterization, micromechanics modeling, and dynamic testing approach. Cem. Concr. Res. 73, 190–206. doi:10.1016/j.cemconres.2015.01.021
CrossRef Full Text | Google Scholar
Mei-Ling, Z., Chuanzhi, S., Li, G., Qiao, Y., Chen, J., Zhang, W., et al. (2022). Investigation on drift ratio limits of PVA fiber reinforced concrete columns under different performance levels based on the Kunnath damage model. Case Stud. Constr. Mater. 17, e01403. doi:10.1016/j.cscm.2022.e01403
CrossRef Full Text | Google Scholar
Minet, J., Abramson, S., Bresson, B., Franceschini, A., Van Damme, H., and Lequeux, N. (2006). Organic calcium silicate hydrate hybrids:a new approach to cement based nanocomposites. J. Mater. Chem. An Interdiscip. J. Deal. Synthesis 16 (14), 1379. doi:10.1039/b515947d
CrossRef Full Text | Google Scholar
Qiu, J., and LimYang, X.-N. E.-H. (2016). Fatigue-induced deterioration of the interface between micro-polyvinyl alcohol (PVA) fiber and cement matrix. Cem. Concr. Res. 90, 127–136. doi:10.1016/j.cemconres.2016.08.021
CrossRef Full Text | Google Scholar
Quan, L., Gongying, D., Zeyu, Z., Fu, C., and Oeser, M. (2021). Investigation on bitumen-epoxy interface in cold mixed epoxy bitumen using experimental observation and molecular dynamics simulation. Constr. Build. Mater. 303, 124490. doi:10.1016/j.conbuildmat.2021.124490
CrossRef Full Text | Google Scholar
Sagar, R. V., and PrasadKumar, B. K. R. S. S. (2012). An experimental study on cracking evolution in concrete and cement mortar by the b -value analysis of acoustic emission technique. Cem. Concr. Res. 42 (8), 1094–1104. doi:10.1016/j.cemconres.2012.05.003
CrossRef Full Text | Google Scholar
Said, M., Montaser, W., Elgammal, A. S., Zahir, A. H., and Shaaban, I. G. (2021). Shear strength of reinforced mortar beams containing polyvinyl alcohol fibre (PVA). Int. J. Civ. Eng. 19 (10), 1155–1178. doi:10.1007/s40999-021-00628-6
CrossRef Full Text | Google Scholar
Tu, Y. M., Shi, P., Liu, D. Y., Wen, R., Yu, Q., Sas, G., et al. (2022). Mechanical properties of calcium silicate hydrate under uniaxial and biaxial strain conditions: a molecular dynamics study. Phys. Chem. Chem. Phys. 24 (2), 1156–1166. doi:10.1039/d1cp04474e
PubMed Abstract | CrossRef Full Text | Google Scholar
Wang, F., and YangYin, Y. S. (2021). Thermal and moisture performance parameters of high toughness engineered cementitious Composite(ECC) with PVA fibers. J. Build. Eng. 43, 102905. doi:10.1016/j.jobe.2021.102905
CrossRef Full Text | Google Scholar
Wang, J., Dai, Q., Si, R., and Guo, S. (2018). Investigation of properties and performances of Polyvinyl Alcohol (PVA) fiber-reinforced rubber concrete. Constr. Build. Mater. 193, 631–642. doi:10.1016/j.conbuildmat.2018.11.002
CrossRef Full Text | Google Scholar
Wenping, D., Caiqian, Y., Hans, D. B., Wang, C., and Pan, Y. (2022). Investigation of the flexural behavior of reinforced concrete beams strengthened with a composite reinforcement layer: polyvinyl alcohol fiber-reinforced ferrocement cementitious composite and steel wire mesh. Struct. Concr. 24 (1), 1612–1626. doi:10.1002/suco.202100894
CrossRef Full Text | Google Scholar
XuWang, G. H. (2018). Diffusion and interaction mechanism of rejuvenating agent with virgin and recycled asphalt binder: a molecular dynamics study. Mol. Simul. 44 (17), 1433–1443. doi:10.1080/08927022.2018.1515483
CrossRef Full Text | Google Scholar
Yang, Y., and Cao, J. (2021). Interfacial heat transfer behavior of graphene-based filler and calcium-silicate-hydrate in cement composites. Int. J. HEAT MASS Transf., 176. doi:10.1016/J.IJHEATMASSTRANSFER.2021.121165
CrossRef Full Text | Google Scholar
Yong, F., Caihua, Y., Kui, H., Yujing, C., Yu, L., and Taoli, Z. (2021). A study of the microscopic interaction mechanism of styrene-butadiene-styrene modified asphalt based on density functional theory. Mol. Simul. 49, 1203–1214. doi:10.1080/08927022.2021.1876874
CrossRef Full Text | Google Scholar
Yong, F., and DajingChen, Q. Z. (2023). The synergistic strengthening effect of silane coupling agent on the interface between PVA/EPS and cement: experiment and molecular simulation. Compos. Interfaces 30 (1), 21–41. doi:10.1080/09276440.2022.2109788
CrossRef Full Text | Google Scholar
Zhou, C., Shi, S. Q., Chen, Z., Cai, L., and Smith, L. (2018). Comparative environmental life cycle assessment of fiber reinforced cement panel between kenaf and glass fibers. J. Clean. Prod. 200, 196–204. doi:10.1016/j.jclepro.2018.07.200
CrossRef Full Text | Google Scholar
Zhuang, L., Robert, W., Fen, D., et al. (2020). Measurement of stress-time avalanches inside polypropylene fiber reinforced concrete beams during flexure. Constr. Build. Mater. doi:10.1016/J.CONBUILDMAT.2020.121428
CrossRef Full Text | Google Scholar
Keywords: fiber-cement composites, silane coupling agent, mechanical properties, molecular dynamics, impact mechanism
Citation: Zhao C, Li L, Li X, Chen W and Li Y (2023) Multi-scale analysis of the synergistic strengthening effect of silane coupling agent on PVA and cement interface. Front. Mater. 10:1276785. doi: 10.3389/fmats.2023.1276785
Received: 13 August 2023; Accepted: 09 October 2023; Published: 19 October 2023.
Copyright © 2023 Zhao, Li, Li, Chen and Li. This is an open-access article distributed under the terms of the Creative Commons Attribution License (CC BY). The use, distribution or reproduction in other forums is permitted, provided the original author(s) and the copyright owner(s) are credited and that the original publication in this journal is cited, in accordance with accepted academic practice. No use, distribution or reproduction is permitted which does not comply with these terms.
*Correspondence: Xiaoyang Li, 2021930676@stu.haut.edu.cn

Alpha Lipoic Acid 600 Physico-Mechanical Properties and Treatment Technology of Hazardous Geomaterials, volume II