Citation numbers are available from Dimensions
University of Agronomic Sciences and Veterinary Medicine, Romania Room Cooler Working Principle

Faculty of Food Engineering, Campinas State University, Brazil
The present study proposes a rapid cooling method based on vacuum spray cooling, and establishes an experimental system to study the cooling performance of this method by taking tofu as an example. In this study, the effects of vacuum spray cooling, vacuum cooling and immersion vacuum cooling on the cooling rate, water holding rate, PH, TPA and other properties of tofu were compared. The total cooling time of vacuum spray cooling (13.86 min) was shorter than that of immersion vacuum cooling (33.39 min) but longer than that of vacuum cooling (10.64 min) for a temperature decrease from 70°C to 4°C. For weight loss from 70°C to 4°C, vacuum spray cooling (2.96%) was significantly less than that of vacuum cooling (10.21%). The PH value after cooling has no significant difference, but the color difference and water holding capacity of the sample after vacuum spray cooling are obviously better. However, the textural properties of the sample cooled by vacuum spray cooling were close to (for elasticity and viscosity) and better (for hardness and chewiness) than those of immersion vacuum cooling. In addition, compared with the two cooling methods in terms of storage, vacuum spray cooling can effectively maintain the moisture content, water holding rate, PH, chromatism and TPA of tofu, thus extending the fresh-keeping period of tofu. The conclusions of this paper provide theoretical support for prolonging the preservation period of food and optimizing the cooling process.
Cooling is regarded as an effective method to improve the shelf life of food with high moisture content (Ditta et al., 2022; Zhang et al., 2022). Vacuum cooling (VC) has the advantages of fast cooling speed (Zhu et al., 2019a), uniform cooling temperature (Dong et al., 2012; Ding et al., 2016), low operating cost and low failure rate (Yesil et al., 2017; Kongwong et al., 2019) which has been widely used in fast food (Zhu et al., 2019b), distribution centecr (Feng and Li, 2015), central kitchen (Ozturk and Hepbasli, 2017) and other industries (Feng et al., 2016). During vacuum cooling, food matrix is placed in a closed cooling oven where the vacuum degree is controlled by the vacuum pump. The boiling point temperature of water can be reduced by increasing the vacuum in the drying chamber, so that the water in the object can evaporate at a lower temperature. However, a degraded in food quality (Liao and Yu, 2020; Song et al., 2020) and the restrictions on samples that could not be cooled is observed after VC which severely limits the promotion of vacuum cooling technology (Rodrigues et al., 2013). Therefore, it is crucial to identify solutions that can reduce the quality loss of food after vacuum cooling.
An important way to reduce the cooling loss is to control the process parameters in the vacuum cooling process. The optimization of vacuum cooling process parameters and the reduction of pressure drop have been extensively studied in the existing articles (Liu et al., 2014; Ozturk et al., 2017). However, the weight loss increases as the final pressure decreases and the final cooling pressure will affect the final cooling temperature in a short cooling time (Ozturk et al., 2011; Santana et al., 2018). Another important factor affecting mass loss is the pressure drop rate. Through the study of flowers and meat, Brosnan and Sun (2003) and Huber and Laurindo (2005) found that with the decrease of the pressure rate, the amount of the mass loss increased significantly. Reducing the volume displacement of the pump can improve the temperature uniformity and reduce mass loss and energy consumption (Song and Liu, 2014; Song et al., 2015). Additionally, process parameters such as loading capacity (Schmidt and Laurindo, 2014; Ding et al., 2018; Santana et al., 2018), product size and shape (Zhang et al., 2013), initial temperature, and final cooling temperature also affect the mass loss in products after vacuum cooling. However, the quality loss in the vacuum cooling process is mainly because of the loss of water in the product. The problem of quality loss cannot be fundamentally solved by simply adjusting the process parameters.
Immersion vacuum cooling (IVC) which immerses a hot-cooked food in a surrounding liquid can achieve a high cooling rate with a comparably lower cooling loss compared to vacuum cooling. It is currently widely used for vacuum cooling of cooked meat and the cooling products obtained by this cooling method have good physical and chemical properties. Drummond and Sun (2012); Feng and Li (2015) found that IVC can effectively improve the quality of meat products and reduce the microbial community in meat products; Drummond et al. (2015) found that IVC can effectively inhibit the growth of spores in pork ham and significantly shorten the cooling time. However, the slow cooling rate of IVC in the later stage of cooling due to the influence of product size hindered its development (Drummond and Sun, 2008). The process of immersion vacuum cooling has been improved to reduce the cooling time by employing appropriate pressure drop rates and increasing stirring (Feng et al., 2013; Feng et al., 2014). In addition, bubble vacuum cooling (Guo et al., 2018; Song et al., 2018), pulse vacuum cooling (Cheng and Sun, 2006), ultrasonic-assisted immersion vacuum cooling (Liao and Yu, 2020) and combined cooling (Cheng and Sun, 2010) are also introduced to improve the cooling rate. However, the cooling time in immersion vacuum cooling cannot be effectively shortened by improving the process. It still has problems such as large consumption of cooling water and large pressure energy loss, which is not conducive to large-scale industrial production.
Because of the spray cooling is characterized by low cooling water volume and fast cooling rate, a new vacuum spray cooling (VSC) method was proposed to compensate for the weight loss in the vacuum cooling process and the longer cooling time in the immersion vacuum cooling process. Based on the current research on cooling, there is little information about the product quality of the tofu cooling process. In this paper, the cooling performance of tofu was studied experimentally including the cooling characteristic, water retention rate, PH value, TPA and the quality change of tofu during storage by three different cooling methods. The research conclusion aims to determine the best cooling method and provide technical support for improving product quality.
Figure 1 shows the schematic diagram of vacuum spray cooling equipment. The spray device has been especially added to the original vacuum cooling equipment (Shanghai Pudong Freezing Dryer Instruments Co., Ltd., Shanghai, China). A thin film of water can be formed on the surface of the food by spraying a small amount of water which can significantly reduce the mass loss. The main components of the spray device are nozzles as shown in Figure 2A (60°S0.4, Danfoss, Denmark), solenoid valves, pressure sensors (SUP-P300, Hangzhou Meiyi Automation Co., Ltd., Hangzhou, China) and temperature sensors. The liquid supply system consists of a micropump (GAV-21, Baizhiyuan Technology Co., LTD, Shenzhen, China), a glass rotameter (LZB-3WB, Changzhou Shuanghuan Thermal Instrument Co., LTD., Jiangsu, China), and a water purifier (HAT-002-Q, Shenzhen Huiante Import and Export Co., LTD., Shenzhen, China). The experimental process of vacuum spray cooling of tofu is demonstrated in Figure 2B.
FIGURE 1. Schematic diagram of a vacuum spray cooling equipment.
FIGURE 2. Diagram of vacuum spray cooling device (A) and process (B).
Fresh tofu was purchased from the Dennis supermarket (Zhengzhou, China) and the samples were wrapped in cling film. Tofu samples were cut into 3 cm squares with a knife immediately upon arrival at the laboratory. The cut samples were stored in a refrigerator (BCD-181LFC, Hangzhou Huari Electric Co., Ltd., Hangzhou, China) at a temperature of 4°C ± 0.5°C. The water used in the experiments was pure water.
Before cooling, all samples were heated in a water bath (DC-3010; Jiangsu Tenlin Instrument Co., Ltd., Jiangsu, China). The temperature of the water bath was set to 80 °C. Once the tofu cores had been at a temperature of 70°C for 2 min, vacuum spray cooling, vacuum cooling and immersion vacuum cooling were performed, respectively. During the vacuum spray cooling process, a number of heated tofu blocks are placed into the vacuum spray cooling equipment. The vacuum pump is switched on and when the vacuum chamber pressure drops to 25 kPa, the vacuum spraying begins. The spray height is 5 cm, the flow rate is 5 ml/min, the spray water temperature is 26°C and the cooling pressure is 700 Pa. In vacuum cooling, tofu blocks are used with a cooling pressure of 700 Pa. In the immersion vacuum cooling process, the heated tofu blocks are first placed in a 1,000 ml beaker and covered with pure water maintained at a temperature of 15°C. The tofu block is then placed into a vacuum chamber with a cooling pressure of 700 Pa.
In the experiment, a thermocouple is inserted from the centre of the side surface of the tofu to its geometric centre. When the temperature in the centre reached 4°C, the experiment ended and the data was recorded. All experiments were repeated three times. At the end of the experiment, the water was removed from the surface of the tofu using filter paper and the tofu samples were then stored in a refrigerator.
Weight loss is defined as:
where X1 (kg) is the weight of the sample before cooling, X2 (kg) is the weight after cooling.
For the purpose of calculating the water holding capacity, 10 g of the sample was placed in the centrifuge tube (SN-SLC-40, Shanghai Sun Power Instrument Co., Ltd., Shanghai, China) at a rotation speed of about 4000R/min. After centrifugation for 30 min, the sample was removed. The centrifuged water was also removed with absorbent paper. The water holding capacity was calculated as:
where A1 (kg) is the weight of the sample before centrifugation, A2 (kg) is the weight after centrifugation.
For PH analysis, 10 g of the sample were mixed with 90 mL of distilled water for 10 min. A digital PH meter (BPH-7100A, Bell Analytical Instruments (Dalian) Co., Ltd., Dalian, China) was used to measure the supernatant.
According to the National Food Safety Standard (GB 5009.3–2010), the moisture content was determined by the direct drying method. Out of the cooled tofu sample, 10 g of tofu was dried in an oven (105 °C) for 24 h. The instrument used for moisture analysis was a rapid halogen moisture tester (FBS-750A, Furbs Ltd., China).
Three pieces of cooled tofu (length * width * height: 3 × 3 × 3 cm) were used for texture profile (TA,XTC, Shanghai Bosin Industrial Development Co., Ltd., Shanghai, China) and color analysis (YS-3060, Shenzhen 3nh Technology Co., Ltd., Shenzhen, China). For evaluating texture profile, an aluminum cylindrical plunger (TA/36) was used. Two cycles of 20% compression with a 10 kg load cell (1.0 mm s−1 deformation rate) were applied to this system. The textural attributes were evaluated by recording the hardness, springiness, gumminess, and chewiness. A Tristimulus colorimeter was used to measure the color on which the Commission Internationale de L’éclairage system (CIE, L∗, lightness; a∗, red/green; b∗, yellow/blue) was applied and the ΔE was calculated according to Eq. 3
where L0*, a0* and b0* are color parameters of fresh tofu; L*, a*, b* are the color parameters of cooled tofu.
The analyses of the TPA properties, chromatism, PH, and moisture were carried out in septuplicate at d1, d2, d3, d4, d5, d6, and d7, respectively.
The data for cooling time, weight loss, color, TPA, moisture content were analyzed by Origin (OriginLab, 2017; United States). One-way ANOVA (Duncan, p < 0.05) was used to compare the differences among the data.
Table 1 shows the average cooling time for tofu for the temperature range from 70°C to 10°C and from 10°C to 4°C and the samples obtained after different cooling methods are shown in Figure 3. There was a significant difference (p < 0.05) in the cooling time and weight loss of the tofu samples obtained by the different cooling methods. The average cooling time for VSC (13.86 min) was shorter than IVC (33.39 min). The cooling time for VSC (7.83 min) was significantly less than that of IVC (16.75 min) in the temperature range from 70°C to 10°C. For the temperature decreased from 10°C to 4°C, the cooling time for VSC (6.03 min) was markedly less than that of IVC (16.64 min). VC exhibited the shortest cooling time because the water inside the tofu evaporated more quickly in a low-pressure environment. IVC immerses tofu in a liquid, whereas VSC forms a thin film of water on the surface of the tofu. Heat transfer takes place mainly by convection, with the cooling rate decreasing later in the cooling process. VSC has less resistance to heat transfer than IVC, which results in faster cooling rates with VSC. A faster cooling method should be chosen to ensure freshness of the tofu during the cooling process.
TABLE 1. Cooling time and weight loss in tofu.
FIGURE 3. Cooled tofu samples obtained by different cooling methods (A) VC (B) VSC (C) IVC.
In addition, large weight losses should be avoided in order to save costs. According to Table 1; Figure 3, the weight loss of the samples from VC (approx. 10.21%) was more than 3.45 times that of VSC (approx. 2.96%). Surprisingly, the weight of the samples after IVC increased by approximately 2.3%, which is significantly heavier than the weight of the IVC. This was due to the penetration of the coolant into the pores and cracks of the foodstuffs during the later stages of IVC. This finding is consistent with the study by Fu et al. (2022) on beverages.
Water retention refers to the ability of food to retain water during mechanical movement. Changes in water retention capacity may be due to changes in the water content and internal structure of the tofu, with the reduced water retention capacity making the tofu more susceptible to mechanical damage. Table 2 shows the changes in water retention before and after cooling with different cooling methods. There was a significant difference in the water holding capacity of the tofu samples obtained by the different cooling methods (p < 0.05) and no significant difference in pH. After VC, the water retention increased from 85.36 to 89.11. After VSC, the water retention increased from 85.88 to 90.17. However, after IVC, the water retention decreased from 85.62 to 83.69. It is clear that tofu treated with VSC is less susceptible to mechanical damage. pH will significantly affect parameters such as microbial growth and enzyme activity, which will have an impact on the quality of the product. Impact. The different cooling methods had no significant effect on the pH of the tofu before and after cooling, while the VSC treatment showed the least difference in pH.
TABLE 2. Water holding rate and PH value for tofu.
Chromatism plays an important role in product quality, especially in foods stored for sale. The experimental data in Table 3 shows that the L* values of the samples cooled by vacuum spray were close to those before cooling. Significant differences (p < 0.05) in L*, a*, b* and ΔE of tofu samples obtained by different cooling methods. The variation in L* values may be due to water loss during the vacuum cooling process. The samples cooled by VSC and IVC have similar a* values compared to before cooling, which is due to the compensation of water in the coolant during the VSC and IVC processes. For the same reason, the b* values of the samples cooled by VSC and IVC are also similar to each other. It is clear that the ΔE for VSC is significantly lower than for the other cooling methods.
TABLE 3. Color change before and after cooling.
The texture of food products is an important indicator of customer purchase intention. Therefore, the texture characteristics of the samples were measured before and after cooling, including hardness, viscosity, elasticity and chewiness. As shown in Table 4, significant differences (p < 0.05) in the hardness, viscosity and chewiness of the tofu samples obtained by the different cooling methods, no significant differences in elasticity. The hardness values of the samples cooled by IVC (∼822.366 gf) were significantly lower than those before cooling (∼876.131 gf). However, the hardness of the samples cooled by VC (∼1,036.586 gf) and VSC (∼959.487 gf) increased. The viscosity of the samples cooled by VC (∼29.20 gf*s), VSC (∼29.29 gf*s) and IVC (∼23.22 gf*s) was remarkably lower than before cooling (∼51.21 gf). For these three cooling methods, the elasticity values did not change much. The textural properties of chewiness showed a similar variation to that of hardness. The quality of the tofu processed with VSC was in a moderate state of softness and hardness, so this was the most suitable cooling method.
TABLE 4. Texture changes for tofu before and after cooling.
Moisture content is an important parameter that may affect other quality parameters including hardness, tenderness, water holding capacity and water activity. Figure 4 shows the changes in moisture during storage under different cooling methods. Under VC and VSC methods, the moisture content decreases significantly on the third day. Under IVC, the moisture content decreases obviously from the second day onwards. The moisture tends to decrease as the storage time increases and the product cooled by the VSC method maintains the highest moisture content. The loss of moisture content may be due to differences in water vapour pressure and surrounding cold air. The increase in moisture content is due to the hydrolysis of the proteins during storage. VC and VSC showed an increase in water content on the fourth day, while IVC showed an increase in water content on the third day, indicating that VC and VSC of the tofu inhibited the growth of microorganisms to some extent.
FIGURE 4. Changes of moisture content of tofu during storage with different cooling methods.
PH is an important factor that may affect other parameters such as elasticity and also has a negative correlation with red colour. As shown in Figure 5, the PH of the food products as a whole decreased during storage. PH values increased significantly on the fourth day after vacuum cooling and vacuum spray cooling, while PH values increased significantly on the third day after immersion vacuum cooling. The VSC-treated tofu had the best ability to maintain PH throughout storage. Fluctuations in PH during storage may be due to changes in water and amine content. The lower levels of ammonia and higher levels of organic acids could be responsible for the decrease in PH at the end of the storage period.
FIGURE 5. PH changes of tofu during storage with different cooling methods.
Water holding capacity is the ability of a food to hold water under the influence of mechanical movement on the water content of the food and changes in the structure of the food. Figure 6 shows the changes in water holding capacity of the samples during storage with different cooling methods. It can be seen that tofu cooled with IVC and VC showed a decreasing trend in water holding capacity during storage. After VSC cooling, the water holding capacity of tofu was significantly better than the other two different cooling methods, allowing for better product quality maintenance. Throughout the storage period, the water holding capacity tends to decrease slowly but fluctuates. This is due to the fact that water retention is influenced by the moisture content of the tofu. In addition, tofu undergoes sugar-acid conversion and spoilage during storage, resulting in an overall decrease in water-holding capacity throughout the storage period.
FIGURE 6. Changes of water holding capacity of tofu during storage with different cooling methods.
An important indicator to evaluate the quality of food during storage is the colour difference, which can directly influence the consumer’s willingness to consume. As shown in Figure 7, the overall colour difference first decreased and then increased after cooling with the different cooling methods. As can be seen in Figure 8, tofu that has undergone VSC retains the best colour compared to the other two methods. In vacuum cooling, the surface of the tofu became yellow and dark as it lost moisture. This colour difference was more pronounced than the other two cooling methods. As storage time increases, the water in the tofu migrates to the outer surface, allowing the proteins to dissolve in the water again, thus reducing the colour difference. After 3–4 days storage, the large number of microorganisms growing and multiplying in the water accelerates the decay of the tofu, resulting in a rapid increase in the yellow colour of the surface.
FIGURE 7. Color difference in tofu during storage with different cooling methods.
FIGURE 8. Chromatism of cooled tofu samples obtained by different cooling methods after 7ds storage. (A) VC (B) VSC (C) IVC.
Textural profile analysis plays an important role in assessing the quality of the product. Figure 9 shows the change in hardness of tofu during storage for different cooling methods. As can be seen from the graph, the hardness of the tofu initially increases and then decreases during storage. The hardness of tofu cooled with VSC is the lowest. As the storage period increases, the amount of water evaporating from within the tofu increases, resulting in an increase in hardness. The later decrease in hardness is due to an increase in the water content of the tofu, leading to hydrolysis of proteins and microbial deterioration. Figure 10 shows the change in viscosity of tofu with different cooling methods during storage. The viscosity of tofu cooled with the different cooling methods shows an initial slow decrease followed by a rapid increase, with the viscosity of tofu cooled with VSC being the most appropriate. The viscosity of tofu is mainly determined by the fat content and protein on the surface of the tofu. As storage time increases, the internal moisture of the tofu evaporates and the moisture content decreases. However, as the internal moisture of tofu migrates, the surface moisture content increases and viscosity decreases. The viscosity then shows a rapid increase, which is caused by the deterioration of the tofu in the presence of microorganisms and the appearance of a sticky substance on the surface of the tofu.
FIGURE 9. Changes of tofu hardness during storage with different cooling methods.
FIGURE 10. Changes of tofu viscosity during storage with different cooling methods.
As shown in Figure 11, the elasticity tended to decrease throughout the storage period, with the VSC-treated sample having the best elasticity. The decrease in elasticity of the tofu indicates that the internal structure of the tofu has softened and the degree of deterioration of the tofu is increasing. Chewiness is the amount of energy required to chew solid food. Figure 12 shows the variation in chewing power between different cooling methods and storage periods. The chewiness of tofu increased and then decreased during storage, while the chewiness of IVC-treated tofu was the lowest. The reason for the difference in chewiness is the evaporation of water during storage. As storage time increases, the chewiness of the different cooling methods tends to increase and then decrease, mainly because the internal water content of the tofu evaporates throughout storage, leading to an increase in chewiness, while as microorganisms hydrolyse the proteins and the tofu itself deteriorates, the internal water content increases and the structure becomes flabby, leading to a decrease in chewiness.
FIGURE 11. Changes of tofu elasticity during storage with different cooling methods.
FIGURE 12. Changes of chewiness of tofu during storage with different cooling methods.
In summary, this paper proposes a vacuum spray cooling (VSC) method to achieve rapid cooling. In order to explore the cooling performance of the VSC-based rapid cooling system, experiments were conducted with tofu as an example. The superiority of VSC compared to conventional tofu cooling methods was demonstrated.
1. Compared to IVC and VC, VSC can significantly reduce the quality loss during vacuum cooling and effectively shorten the cooling time. Notably, the water holding capacity and pH value of tofu can be well maintained after the VSC treatment.
2. The colour difference of the VSC-treated tofu samples is minimal. The hardness and chewiness values of the samples cooled by IVC were significantly lower than before cooling. However, the hardness and chewiness of the samples cooled by VC and VSC increased. The viscosity of the cooled samples was significantly lower than the viscosity before cooling. There was no significant effect of the three cooling methods on the elasticity values. The quality of the tofu processed with VSC was in a medium state.
3. After a period of storage, the VSC-treated tofu samples had the best water content, pH value, water holding capacity and colour. Based on TPA testing, it was found that the VSC-treated samples retained the best hardness, visibility, elasticity and good chewiness.
The results of this study provide a theoretical reference for improving the cooling efficiency and quality of tofu. In addition, proper pre-treatment before cooling can also improve cooling speed and product quality. In the next research work, we will further analyse technical solutions to reduce cooling consumption and improve tofu quality in conjunction with pretreatment techniques.
The original contributions presented in the study are included in the article/Supplementary material, further inquiries can be directed to the corresponding author.
GL: investigation, methodology, LH and SD: data curation, software, and writing—original draft. YP and ZZ: investigation, validation, and formal analysis. CC: supervision, investigation. TJ: funding acquisition. All authors contributed to the article and approved the submitted version.
This work was supported by the Postgraduate education innovation training base project of Henan Province (Grant No. YJS2021JD05) and the Henan Provincial Department of Science and Technology Research Project (Grant No. 222102320075).
The authors declare that the research was conducted in the absence of any commercial or financial relationships that could be construed as a potential conflict of interest.
All claims expressed in this article are solely those of the authors and do not necessarily represent those of their affiliated organizations, or those of the publisher, the editors and the reviewers. Any product that may be evaluated in this article, or claim that may be made by its manufacturer, is not guaranteed or endorsed by the publisher.
Brosnan, T., and Sun, D. W. (2003). Influence of modulated vacuum cooling on the cooling rate, mass loss and vase life of cut lily flowers. Biosyst. Eng. 86 (1), 45–49. doi:10.1016/S1537-5110(03)00101-6
CrossRef Full Text | Google Scholar
Cheng, Q., and Sun, D. W. (2010). Effects of combined water cooking–vacuum cooling with water on processing time, mass loss and quality of large pork ham [J]. J. Food Process Eng. 30 (1), 51–73. doi:10.1111/j.1745-4530.2007.00089.x
CrossRef Full Text | Google Scholar
Cheng, Q., and Sun, D. W. (2006). Improving the quality of pork ham by pulsed vacuum cooling in water [J]. J. Food Process Eng. 29 (2), 119–133. doi:10.1111/j.1745-4530.2006.00052.x
CrossRef Full Text | Google Scholar
Ding, T., Gobber, C., Santana, J. C. C., Alves, W. A. L., Araújo, S. A. d., and Dong-Hong, L. (2018). Combination of optimization techniques to find of processing optimal condition of postharvest broccoli by vacuum cooling process. Acta sci-technol. 40, e36222. doi:10.4025/actascitechnol.v40i1.36222
CrossRef Full Text | Google Scholar
Ding, T., Liu, F., Ling, J. D., et al. (2016). Comparison of different cooling methods for extending shelf life of postharvest broccoli [J]. Int. J. Agr. Biol. Eng. 9 (6), 178–185. doi:10.3965/j.ijabe.20160906.2107
CrossRef Full Text | Google Scholar
Ditta, A., Tabish, A. N., Abbas, M. A., et al. (2022). Experimental investigation of hybrid configuration of solar thermal collectors and desiccant indirect evaporative cooling system[J]. Front. Energy Res. 10, 1432. doi:10.3389/fenrg.2022.979942
CrossRef Full Text | Google Scholar
Dong, X., Chen, H., Liu, Y., Dai, R., and Li, X. (2012). Feasibility assessment of vacuum cooling followed by immersion vacuum cooling on water-cooked pork. Meat Sci. 90 (1), 199–203. doi:10.1016/j.meatsci.2011.07.002
PubMed Abstract | CrossRef Full Text | Google Scholar
Drummond, L., Meinert, L., Koch, A. G., Würtz, J., Zhang, Z., and Sun, D. W. (2015). Safety and quality evaluation of large meat joints cooled by a precommercial immersion vacuum cooling prototype. Int. J. Food Sci. Nutr. 50 (9), 2066–2073. doi:10.1111/ijfs.12867
CrossRef Full Text | Google Scholar
Drummond, L., and Sun, D. W. (2012). Evaluation of the immersion vacuum cooling of cooked beef joints—Mathematical simulation of variations in beef size and porosity and pressure reduction rates. Innov. Food Sci. Emerg. 16, 205–210. doi:10.1016/j.ifset.2012.06.001
CrossRef Full Text | Google Scholar
Drummond, L., and Sun, D. W. (2008). Immersion vacuum cooling of cooked beef - Safety and process considerations regarding beef joint size. Meat Sci. 80 (3), 738–743. doi:10.1016/j.meatsci.2008.03.014
PubMed Abstract | CrossRef Full Text | Google Scholar
Feng, C. H., Drummond, L., Zhang, Z. H., and Sun, D. W. (2013). Effects of processing parameters on immersion vacuum cooling time and physico-chemical properties of pork hams. Meat Sci. 95 (2), 425–432. doi:10.1016/j.meatsci.2013.04.057
PubMed Abstract | CrossRef Full Text | Google Scholar
Feng, C. H., Drummond, L., Zhang, Z. H., and Sun, D. W. (2014). Evaluation of innovative immersion vacuum cooling with different pressure reduction rates and agitation for cooked sausages stuffed in natural or artificial casing. Lwt-Food Sci. Technol. 59 (1), 77–85. doi:10.1016/j.lwt.2014.04.035
CrossRef Full Text | Google Scholar
Feng, C. H., García Martín, J. F., Li, C., Liu, B. L., Song, X. Y., Dong, Q. L., et al. (2016). Evaluation of physicochemical properties and microbial attributes of cooked sausages stuffed in casing modified by surfactants and lactic acid after immersion vacuum cooling and long-term storage. Int. J. Food Sci. Tech. 51 (10), 2270–2279. doi:10.1111/ijfs.13224
CrossRef Full Text | Google Scholar
Feng, C., and Li, C. (2015). Immersion vacuum-cooling as a novel technique for cooling meat products: Research advances and current state-of-the art. Compr. Rev. Food Sci. F. 14 (6), 785–795. doi:10.1111/1541-4337.12157
CrossRef Full Text | Google Scholar
Fu, H., Zhao, R., Long, W., and Cheng, W. (2022). Study on cooling performance of rapid cooling system based on vacuum spray flash evaporation. Appl. Therm. Eng. 201, 117751. doi:10.1016/j.applthermaleng.2021.117751
CrossRef Full Text | Google Scholar
Guo, Z. Y., Song, X. Y., Song, Z., and Liu, B. L. (2018). An improved method of immersion vacuum cooling for small cooked pork: Bubbling Vacuum Cooling. Int. J. Food Sci. Tech. 53 (12), 2748–2753. doi:10.1111/ijfs.13918
CrossRef Full Text | Google Scholar
Huber, E., and Laurindo, J. B. (2005). Weight loss of precooked chicken breast cooled by vacuum application [J]. J. Food Process Eng. 28 (3), 299–312. doi:10.1111/j.1745-4530.2005.00419.x
CrossRef Full Text | Google Scholar
Kongwong, P., Boonyakiat, D., and Poonlarp, P. (2019). Extending the shelf life and qualities of baby cos lettuce using commercial precooling systems. Postharvest Biol. Tec. 150, 60–70. doi:10.1016/j.postharvbio.2018.12.012
CrossRef Full Text | Google Scholar
Liao, C., and Yu, Y. (2020). An innovative method of immersion vacuum cooling for cooked meat products: Immersion vacuum cooling with ultrasonic assistance. Int. J. Food Sci. Tech. 55 (7), 2771–2782. doi:10.1111/ijfs.14530
CrossRef Full Text | Google Scholar
Liu, E., Hu, X., and Liu, S. (2014). Experimental study on effect of vacuum pre-cooling for post-harvest leaf lettuce. J. Food Nutr. Res-Slov. 2 (8), 907–449. doi:10.5958/2348-7542.2014.01429.6
CrossRef Full Text | Google Scholar
Ozturk, H. M., Ozturk, H. K., and Kocar, G. (2011). Comparison of vacuum cooling with conventional cooling for purslane [J]. Int. J. Food Eng. 7 (6), 123–134. doi:10.2202/1556-3758.2442
CrossRef Full Text | Google Scholar
Ozturk, H. M., Ozturk, H. K., and Koçar, G. (2017). Microbial analysis of meatballs cooled with vacuum and conventional cooling. J. Food Sci. Tech. 54 (9), 2825–2832. doi:10.1007/s13197-017-2720-7
CrossRef Full Text | Google Scholar
Ozturk, H. M., and Hepbasli, A. (2017). Experimental performance assessment of a vacuum cooling system through exergy analysis method. J. Clean. Prod. 161, 781–791. doi:10.1016/j.jclepro.2017.05.118
CrossRef Full Text | Google Scholar
Rodrigues, L. G. G., Cavalheiro, D., Schmidt, F. C., and Laurindo, J. (2013). Possibilities for integrating cooking and vacuum cooling of potatoes in the same vessel [J]. J. Food Process Pres. 37 (5), 846–854. doi:10.1111/j.1745-4549.2012.00719.x
CrossRef Full Text | Google Scholar
Santana, J. C. C., Araújo, S. A., Alves, W. A. L., Belan, P. A., Jiangang, L., Jianchu, C., et al. (2018). Optimization of vacuum cooling treatment of postharvest broccoli using response surface methodology combined with genetic algorithm technique. Comput. Electron Agr. 144, 209–215. doi:10.1016/j.compag.2017.12.010
CrossRef Full Text | Google Scholar
Schmidt, F. C., and Laurindo, J. B. (2014). Alternative processing strategies to reduce the weight loss of cooked chicken breast fillets subjected to vacuum cooling. J. Food Eng. 128, 10–16. doi:10.1016/j.jfoodeng.2013.12.006
CrossRef Full Text | Google Scholar
Song, X., Guo, Z., Liu, B., and Jaganathan, G. K. (2018). Evaluation of bubbling vacuum cooling for the small-size cooked pork. Food Bioprocess Tech. 11 (4), 845–852. doi:10.1007/s11947-018-2058-9
CrossRef Full Text | Google Scholar
Song, X., Liu, B., Jaganathan, G. K., and Chen, L. (2015). Mechanism of spillage and excessive boiling of water during vacuum cooling. Int. J. Refrig 56, 37–42. doi:10.1016/j.ijrefrig.2015.04.009
CrossRef Full Text | Google Scholar
Song, X., and Liu, B. (2014). The optimization of volumetric displacement can uniformize the temperature distribution of heated ham during a vacuum cooling process. Food Sci. Technol. Res. 20 (1), 43–49. doi:10.3136/fstr.20.43
CrossRef Full Text | Google Scholar
Song, X. Y., Song, Z., Liu, B. L., Guo, Z. Y., and Luan, Y. (2020). Effect of vacuum cooling on stability of macro-porous sausage during refrigerated storage-Vacuum-cooled sausage has a longer shelf life. Food Sci. Nutr. 8 (5), 2223–2233. doi:10.1002/fsn3.1435
PubMed Abstract | CrossRef Full Text | Google Scholar
Yesil, M., Kasler, D. R., Huang, E., and Yousef, A. E. (2017). Efficacy of gaseous ozone application during vacuum cooling against Escherichia coli O157:H7 on spinach leaves as influenced by bacterium population size. J. Food Prot. 80 (7), 1066–1071. doi:10.4315/0362-028X.JFP-16-284
PubMed Abstract | CrossRef Full Text | Google Scholar
Zhang, X., Yan, G., Yu, X., Li, H., Zhang, Y., and Song, Y. (2022). Effects of particle diameter and plate thickness on transpiration cooling for double-layer porous plates. Front. Energy Res. 10, 1761. doi:10.3389/fenrg.2022.1050399
CrossRef Full Text | Google Scholar
Zhang, Z., Drummond, L., and Sun, D. W. (2013). Vacuum cooling in bulk of beef pieces of different sizes and shape – evaluation and comparison to conventional cooling methods. J. Food Eng. 116 (2), 581–587. doi:10.1016/j.jfoodeng.2012.12.036
CrossRef Full Text | Google Scholar
Zhu, Z., Geng, Y., and Sun, D. W. (2019a). Effects of operation processes and conditions on enhancing performances of vacuum cooling of foods: A review. Trends Food Sci. Tech. 85, 67–77. doi:10.1016/j.tifs.2018.12.011
CrossRef Full Text | Google Scholar
Zhu, Z., Li, Y., Sun, D. W., and Wang, H. W. (2019b). Developments of mathematical models for simulating vacuum cooling processes for food products - a review. Crit. Rev. Food Sci. 59 (5), 715–727. doi:10.1080/10408398.2018.1490696
CrossRef Full Text | Google Scholar
Keywords: vacuum spray cooling, immersion vacuum cooling, cooling time, TPA, quality
Citation: Li G, Han L, Du S, Peng Y, Zhang Z, Cheng C and Jin T (2023) Experimental investigation of the characteristic of vacuum spray cooling for tofu. Front. Food. Sci. Technol. 3:1191360. doi: 10.3389/frfst.2023.1191360
Received: 22 March 2023; Accepted: 30 June 2023; Published: 14 July 2023.
Copyright © 2023 Li, Han, Du, Peng, Zhang, Cheng and Jin. This is an open-access article distributed under the terms of the Creative Commons Attribution License (CC BY). The use, distribution or reproduction in other forums is permitted, provided the original author(s) and the copyright owner(s) are credited and that the original publication in this journal is cited, in accordance with accepted academic practice. No use, distribution or reproduction is permitted which does not comply with these terms.
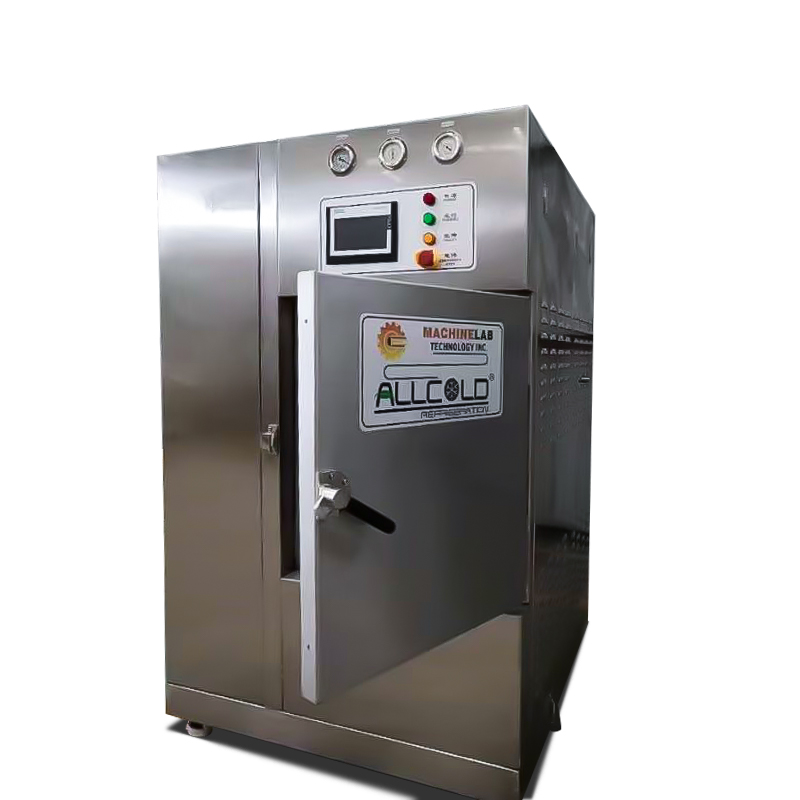
Evaporative Cooler Principle *Correspondence: Tingxiang Jin, txjin@126.com