Thank you for visiting nature.com. You are using a browser version with limited support for CSS. To obtain the best experience, we recommend you use a more up to date browser (or turn off compatibility mode in Internet Explorer). In the meantime, to ensure continued support, we are displaying the site without styles and JavaScript.
Scientific Reports volume 14, Article number: 8648 (2024 ) Cite this article Staple fibers geotextile

Geotextiles made from plant fibers creates a suitable environment for plant growth as part of soil bioengineering techniques. The faster decomposition of plant fiber geotextiles compared to synthetic ones demands the use of composites that enhance their waterproofing and extend their durability in the environment. The objective of this work was to evaluate the resistance of a geotextile made with Thypha domingensis to degradation caused by climatic variables. Tensile strength tests were conducted in the laboratory in order to evaluate the degradation of geotextiles treated with single and double layers of waterproofing resin. Based on Scanning Electron Microscopy (SEM) images, it was verified that applying double layer of waterproofing resin delays the fibers degradation up to 120 days of exposure to the effects of climatic variables other than temperature. The maximum resistance losses due to the geotextile's exposure to degradation were statistically significant for all three treatments: control-without waterproofing resin, with one layer resin, and with two layers resin. Therefore, waterproofing resin, provides a long-term protective solution for geotextiles made from cattail fibers.
Soil erosion is a geomorphic process resulting from soil disruption, transport of particles, rock fragments, organic matter, and siltation of water systems1. Features of topography, geomorphology, vegetation, and anthropic activities are factors that interfere on the stability of slopes and is associated to erosion processes2. Mitigating measures to control erosion on slopes use different techniques, such as soil bioengineering3,4. Soil bioengineering brings together materials and techniques from the field of biology and civil engineering based on the use of inert materials such as steel, wood, rock, and concrete, as well as biological materials such as live cuttings and seedlings of plant species, and also geotextiles5,6. Also soil bioengineering involves techniques that use plants as living building materials to control soil erosion7, torrential floods and landslides and in order to reach ecological restoration or even the reintroduction of species in degraded lands8, offering a range of benefits for humans and nature.
The utilization of natural geotextiles in conjunction with sustainable technologies has demonstrated improvement in soil protection, while also proving to be more economically viable than traditional engineering techniques9. Geotextiles provide direct protection against soil erosion, exhibiting controlled degradation, and help in the process of revegetation, minimizing erosion processes10.
Geosynthetics, such as geotextiles and geomembranes, designed according to the geotechnical land´s needs has become an emerging field in civil engineering and other fields in which they offer varied areas of application11. The main advantages of synthetic products are their slow biodegradability, which leads to greater durability, and their adaptability for specific engineering uses at a reduced cost12. However, low tensile modulus, ease of photodegradation, lower permeability, and restriction to the development of soil flora and fauna are among the main disadvantages of geosynthetics for the protection and recovery of degraded areas13.
The use of geotextiles offers rapid protection against erosive processes in the soil, increases the resistance of the slopes, and promotes the rapid growth of vegetation, all of which are very effective in controlling erosion14. According to Yan et al15., by allowing the development of a vegetation cover, geotextiles create an environment that intercepts the precipitation and reduces splashes due to the different morphologies and structures of the developed vegetation, ultimately controlling soil erosion.
Most of these geotextiles originate from synthetic materials can fail in the field due to detachment or inadequate interaction between the soil and the geosynthetic, which leads to loss of effectiveness16. Additionally, the use of geosynthetics can generate environmental issues such as soil pollution and the accumulation of microplastics17. Thus, it is necessary to choose a product that is efficient and ecologically stable in its long-term properties18.
Vegetable fibers used in geotextile production offer a favorable combination of mechanical and environmental properties 19. These geotextiles present advantages like simplified implementation, compatibility with the surrounding landscape and environment, contributing to the preservation of local biodiversity20. Therefore, comprehensive studies on the durability of natural geotextiles, both in laboratory and field settings, are important to better recognize their properties and ensure successful slope and riverbank stabilization, promoting soil anchorage21.
Some natural geotextiles made from Cocos nucifera L., Agave sisalana Perrine ex Engelm, and Hibiscus canabinus L. fibers have good mechanical properties comparable to synthetic fabrics for flooring and, therefore, may be advantageous for soil bioengineering applications22.
Another species that presents outstanding potential for the manufacture of geotextiles from its fibers is Thypha domingensis, a plant with long green leaves that grows on the banks of lakes and dams, marshes and swamps and can be found throughout tropical and subtropical America, being used for the manufacture of handicrafts23.
T. domingensis, a plant species extensively distributed across the tropical and subtropical regions of the Americas, is commonly found in lake margins, marshes, and wetland areas24. Its widespread availability, coupled with a relatively straightforward process for fiber collection and processing, as well as its ease of cultivation, render it a viable candidate for the production of natural geotextiles25. Furthermore, as a renewable and biodegradable resource, geotextiles derived from T. domingensis are environmentally sustainable and can be utilized in soil stabilization projects without any adverse impacts on the environment26.
Regularly found in wetland ecosystems, its extensive presence in over 80 countries makes it a suitable resource to produce geotextiles on a large scale27. In tropical countries such as Brazil, Mexico, and India, Typha domingensis is notably abundant, often classified as an invasive species due to its rapid growth28.
Like geosynthetics, geotextiles made from natural fibers suffer deterioration from different environmental factors, including friction, moisture, and exposure to UV light. Geotextiles exposed to UV light at wavelengths between 300 and 400 nm are more vulnerable to photo-oxidation 29. In geosynthetics, to avoid its degradation in long-term uses, supplemental additives help the fibers to remain stable for longer30.
The most common protective additives applied in geosynthetics include Chimassorb 944 and carbon black, which helps polymers to resist the UV light damage that accelerates material degradation31. However, little has been explored on the geotextiles manufacture32, related to the development of protective compounds in order to increase the durability of natural fiber geotextiles in the field, and on the complexities of the effects of resistance and durability from the interaction with the environmental variables and the soil, since the mechanisms are commonly measured based on empirical procedures. Although additives delay the effects of degradation, the decomposition of the fibers still happens, with the long-term durability of the product being affected33, thus, it is necessary to verify the durability of the geotextiles both in the field and in laboratory tests to better understand fiber-composite interactions, helping the slope stabilization and potential floristic recovery34.
Therefore, to contribute to the research on the durability of geotextiles composed of natural fibers, the objective of this work was to evaluate the resistance of a geotextile made with T. domingensis to degradation caused by climatic variables.
Samples of T. domingensis (Fig. 1) plants were selected and collected based on the mechanical application of the fiber, cellulosic and lignin content reported in the literature, in 2 (two) municipalities of the Lower São Francisco River stretch (Northeastern Brazil), between 2019 and 2020. T. domingensis is a perennial herbaceous plant of the genus Typha, of the Typhaceae family included in the SisGen database.
Harvesting of T. domingensis for fiber extraction and geotextile production.
After collection, the samples were taken to the laboratory for drying, fraying and separation of the fibers in order to manufacture the geotextiles. The studied species were registered in the Sistema Nacional de Gestão do Patrimônio Genético e do Conhecimento Tradicional Associado (National System for the Management of Genetic Heritage and Associated Traditional Knowledge) with the following registration code SisGen A2B3842.
Obligatory licenses were obtained for the collection of wild plant specimens in Brazil, and the collection protocol was carried out in accordance with the standard procedures recommended by the National System for the Management of Genetic Heritage and Associated Traditional Knowledge “SisGen”. The formal identification of the plant material was made by Dr. Maria Luiza Silveira de Carvalho, professor of Botany at Biology Institute-Universidade Federal da Bahia. Voucher samples were kept in the Herbarium of Universidade Federal de Sergipe (ASE), São Cristóvão-SE, Brazil.
This study was carried out on a steep slope of 18.3° in a soil classified as a Typic Quartzipsamment (10°55′47.2″ S 37°06′12.6″ W), with more than 90% of sand particles in the texture, and very low water retention capacity, strongly influencing in the geotextile degradation rhythm, exposed to climatic variables, from march to august 2021, during the rainy season, with the temperature varying from 21 °C to 29 °C, and total rainfall in the period of 720 mm, located on the Campus of the Universidade Federal de Sergipe, Municipality of São Cristóvão, Sergipe state, in northeastern Brazil. Geotextiles made with T. domingensis fibers measuring 1 m2 were randomly distributed and submitted to the following treatments: (a) geotextile with no waterproofing resin (control); (b) geotextile treated with a single layer of waterproofing resin; and (c) geotextile treated with a double layer of waterproofing resin.
The production process of the T. domingensis geotextile involves carefully planned steps to ensure the quality and effectiveness of the material. Initially, the plant was harvested using appropriate cutting tools to preserve the integrity of the fibers, which is necessary to keep the strength of the geotextile. After harvesting, the fibers was dried in the shade for about six to eight days, and storaged in dried environment.
The thickness and fibers length, which may vary depending on environmental conditions, were meticulously selected in the manufacturing process of the geotextile. The weaving process involves twisting and braiding the fibers on a wooden mold, to provide resistance to traction.
The geotextiles were manufactured from T. domingensis fibers and subjected to treatment with a colorless wood waterproofing resin by Hydronorth©. The selection of a colorless wood waterproofing resin in the geotextile´s production was driven by its specific properties that align with the desired characteristics of the product35. This type of resin provides robust waterproofing capabilities without the addition of harmful chemicals or heavy pigments that could leach into the environment36, thus preserving the biodegradability and ecological compatibility of the geotextile. Additionally, this product was compatible to the available apparatus used to spray over the fibers.
The geotextiles were covered with single and double layers of waterproofing resin and then exposed to the effect of climatic variables degradation on the slope, such as rain, solar radiation, temperature, and wind. The waterproofed material was kept for incubation for approximately 48 h at room temperature before field exposure37. This time length guaranteed suitable fixation and effectiveness of the waterproofing treatment.
Geotextile samples measuring 30 cm in length were collected every 30 days over a period of 180 days. The environmental conditions (temperature, humidity, precipitation, and solar radiation) were monitored daily through the gathering of information conducted by the meteorological station38.
The natural degradation test analyzed the behavior of the geotextile under rain, solar radiation, temperature, wind, etc. and was carried out at different levels of degradation in the field. Six sample collections were performed at 30 days intervals as follows: T1 (30 days), (T2 60 days), (T3 90 days), (T4 120 days), (T5 150 days) and (T6 180 days) of exposure.
Scanning Electron Microscopy (SEM) images were obtained through randomly selected samples at predefined sampling times, and fibers were cut into 1 cm × 1 cm samples for evaluation. The samples were metalized with gold in the Cressington of the manufacturer Kurt J. Lesker 108 of the Laboratory of Corrosion and Nanotechnology (LCNT). The used equipment was a HITACHI scanning electron microscope, model TM 3000, from the Center for Multiuser Chemistry Laboratories (CLQM-UFS), and the images were obtained on the surface of the metallized sample through the scanning of an electron beam of 15 keV and under vacuum39.
Unconfined tensile strength tests were carried out at the Materials Engineering Laboratory of the Universidade Federal de Sergipe (UFS) using an EMIC Model DL universal testing machine with a maximum capacity of 300 KN40. In order to conduct the tensile strength tests of the geotextile fibers, 7 (seven) samples were considered after the field degradation processes. The applied distance between the steel jaws with the inner faces was of 100 mm (Fig. 2), and the deformation speed was of 20 mm/min.
Tensile strength tests of T. domingensis fibers.
To secure the fibers in the universal testing machine, a pair of steel claws was used, with the internal faces contacting the fibers to prevent a possible displacement during the application of traction forces. Load VS curves were performed within the test’s proceeeding. The analyzed characteristics were tensile strength at maximum load (α max), tensile deformation (εii) and secant stiffness (Jsec).
In order to evaluate the interaction between the different treatments and the physical characteristics of the fibers throughout the evaluation period (T1—30th day to T 7—180th day), multivariate analysis of variance for repeated measures (MANOVA-MR) were performed. A regression analysis was also performed to investigate the effect of time on degradation in the mechanical characteristics of resistance of T. domingensis fiber that differed with the application of two levels of waterproofing resin.
Post hoc analyses for main effects and interaction effects (exposure times*waterproofing resin) were performed using the Bonferroni test and considering the effect size (r) by Cohen’s D41 and F-value (F).
To describe the statistical strength of the physical resistance of the geotextile with an application of waterproofing resin, the effect sizes were evaluated by Eta squared (η^2) and Cohen's D were used for analyzes means and differences in means (ΔM). The effect size refers to the power that an independent variable (VI) exerts on a dependent variable (DV). In this study, the size of the obtained effect by the VD characterizes the effect of the geotextile treatment in relation to the control treatments (T1 and without waterproofing resin).
The data normality was assessed through the Kolmogorov‒Smirnov (KS) and Shapiro‒Wilk (SW) residual tests. The variance homogeneity assumption was evaluated through the Levene’s test. The assumption of sphericity was obtained by the Mauchly test, and when not complied with, Greenhouse‒Geisser values for sphericity < 0.75 and Huynh–Feldt for sphericity > 0.75 will be assigned.
Bootstrapping procedures with 1000 resamplings; 95% Bias-Corrected and Accelerated (BCa) Confidence Interval (CI) were implemented to achieve a greater reliability of the results, to correct deviations from normality in the sample distribution and differences between the sizes of the groups, and to present a confidence interval of 95% for differences among the average data42. All statistical tests were performed at p value = 0.05, and mean differences (ΔM) and marginal estimates were performed with confidence interval adjustment throughout the Bonferroni method.
Regression assessments were applied to investigate to what extent the waterproofing agent applied on the fibers under different levels of climatic degradation explains the longevity of its resistance through the physical studied characteristics.
During the samples’ evaluation, the tensile strength was gradually reduced under the effect of climatic variables, promoting the geotextile degradation over time. The normality distribution data of the residuals showed that only treatment T1(30 days) [KS = 0.263, p < 0.001; SW = 0.821, p < 0.001] did not show a normal distribution, and Levene’s test showed that all groups showed homogeneity of variance. Mauchly's sphericity test did not meet the sphericity assumption (Mauchly's W = 0.002 c2 (20) = 98.947, p = 0.001),R and the general result of ANOVA-MR (Since the sphericity test was not conducted, Huynh-Feldt values (> 0.75) were considered for within-subjects effects) showed that there were statistically significant differences in tensile strength over the 180-day experiment (F (4, 62.908) = 9.013, p < 0.001; η2 = 0.255).
The results showed that when exposed to natural degradation, T. domingensis fibers decreases in tensile strength at maximum load throughout the aging process of 180 days. A statistically significant difference was presented by the samples without waterproofing resin (F (1, 45) = 31,386, p < 0.032; R2 = 0.411), marginally significant for the samples with the application of a layer of waterproofing resin (single layer) (F (1, 45) = 22,618, p < 0.045; R2 = 0.682) and not significant for double layer (F (1, 47) = 23,853, R2 = 0.752, p < 0.065). These data demonstrate that the maximum tensile strength of the fibers has low variability over a 180-day exposure to natural degradation but vary with or without waterproofing resin treatments, showing slightly higher in those that received protective treatment with waterproofing resin than the ones without the application of waterproofing resin. Figure 3 shows the behavior on Scanning Electron Microscopy (SEM) images related to treatment with and without waterproofing resin.
SEM images of T. domingensis fibers after 180 days without treatment (a), one layer of waterproofing resin (b), and two layers of waterproofing resin (c).
The images show the SEM results of the geotextile samples that were collected after a 180-day exposure to degradation. The geotextile sample without waterproofing treatment (Fig. 3a) demonstrates that a complex structure of the fiber presents cellulosic loss points of 10.153 µm diameter and 1.581 µm in diameter, as well as other minor degradation points. On the samples of geotextile fibers treated with waterproofing resin with one layer (Fig. 3b), the presence of longitudinal cracks could be noted, even though there were no transverse cracks. Fibers treated with two layers of waterproofing resin (double layer) (Fig. 3c) showed greater material integrity, and fiber breakage, degradation points, or cracks were not observed.
A study performed by Carneiro et al43. using SEM to evaluate the degradation behavior in manufactured geotextiles showed that during exposure to natural light, geotextiles present extensive damage to natural UV light exposure, with many fibers cracked and broken. It is important to note that the previous authors evaluated geotextiles treated with protective stabilizers in their compositions, evaluating damage to geotextiles using SEM techniques. Franco et al33., analyzing the behavior of geotextiles observed that when not treated with UV stabilizers, they present degraded points in a shorter time than those treated with stabilizers. Then it is possible to note that in the absence of any stabilizers, geotextiles are highly susceptible to UV degradation, which explains the observed damage in Fig. 2a and the loss of physical resistance of the material.
Figure 4 presents data from the regression tests for maximum traction (α max). Comparing independent samples of T. domingensis geotextile, it was observed that the losses of maximum resistance due to the exposure of the geotextile to degradation were statistically significant in the three treatments (without resin: F (1, 45) = 31.386, p < 0.001; adjusted R2 = 0.411; single layer: F (1, 45) = 22.618, p < 0.001; adjusted R2 = 0.334; double layer: F (1, 45) = 24.497, p < 0.001; adjusted R2 = 0.338). The data demonstrates that there is a more significant influence to the loss of maximum resistance related to degradation for fibers that are not treated with waterproofing in comparison to the ones that are, a factor that may have generated a greater difference in mean at the end of the experiment for the untreated fibers.
Tensile strength at break (α max) of geotextiles fibers over 180 days.
Natural fibers show reductions in tensile strength due to increased depolymerization at higher temperatures44. Thus, the application of two layers of waterproofing resin may have reduced the effective temperature in the fibers as a result of the reduction in direct contact of the fibers with the heated soil during the exposure time. Das et al. observed that the amount of lignin present in coconut fibers (C. nucifera) reduced its resistance by 6% when exposed to 1 h of heating at 180 °C. While studying jute fibers (Corchorus capsularis), Khan et al45. noted that fibers exposed to degradation lost 10% of tensile strength when exposed to 170 °C in 1 h since, as the applied temperature increases, there is an increase in creep deformations and a decrease in elastic stiffness in geotextiles non-woven46.
The regression coefficient B for the treatment without waterproofing resin indicates that every 30 days of degradation, the T. domingensis fiber tensile strength decreases by 13.86% (B = − 14.446 N/mm−1, 95% [BCa = − 19.493–− 9.252]). This amount is reduced by 11% with the application of a double layer (B = − 12.408 N/mm−1, 95% [BCa = − 17.057–− 7.359]). Therefore, the treatment with the application of 2 layers of waterproofing resin plays an important role in controlling the residual losses of the tensile strength of this geotextile.
Differences in the tensile strength of the control sample (without waterproofing resin) and the geotextile treated with the resin were also analyzed through cross-sectional statistics between periods.
To understand the best influenced period and the differentiation of treatments' tensile strength losses, post hoc tests were carried out using each treatment longitudinally. The initial time (T1) was considered the control data for each treatment. The results show that the most stable significant differentiation of the natural degradation of the fibers between the treatments occurred after a 150-day exposure (T5). The geotextile was subjected to a 90-day exposure time.
During a 150-day period, the control treatment showed an average tensile strength of 1.899 N/mm−1 and residual strength of R RES = 21.66% (ΔM = 6.822 N/mm−1; p < 0.001, Cohen's D = 1.530); however, when the geotextile was treated with one layer of waterproofing resin, the fibers presented an average of 2.982 N/mm−1 and RRES = 33.66% for the same time (ΔM = 5.871 N/mm−1; p < 0.001, Cohen’s D = 0.606), both showing significant differences.
When the geotextile was submitted to a double waterproofing layer during the same period (T5), the fibers showed marginally significant differences in tensile strength and lower power of effect than the other treatments (ΔM = 5.556 p < 0.055, Cohen’s D = 0.488), with a mean of 4.373 N/mm−1. Residual strength values higher than the other studied treatments were also noted RRES = 55.05% (Table 1).
Similar values of maximum tensile strength were also observed by Basu et al9. for the same period when analyzing geotextiles made from natural fibers reinforced by synthetic fibers. The obtained data demonstrate that the application of two layers of waterproofing resin extends the tensile strength and consequently improves the resistance to friction against the soil, improving the rigidity and textile structure of the blanket47. Carneiro et al48. observed in their study that the successive exposure of non-woven geotextiles to thermal oxidation without protection caused a decrease in tensile strength of up to 30.3%.
The loss of tensile strength (α max) occurs gradually in geotextiles manufactured from natural fibers49. One of the reasons behind the extending of resistance due to the use of protectors or waterproofing agents can be attributed to the reduction in the porosity of the material, which reduces the water infiltration, thus hindering microbial degradation. Pore reduction allows for the prolonged maintenance of key geotechnical properties50.
The stress–strain curve depicted in Fig. 5 illustrates a decline in tensile strength (α max) in the end of the sampling time (180 days). Such response is typical of materials that exhibit elastic behavior, such as natural fibers. For the untreated fiber, linearity is maintained until a yield limit is reached, which in this work is approximately 13.42 M. Beyond this point, the material no longer deforms elastically but enters a phase of plastic deformation, marking the onset of permanent deformation—a phenomenon similar to that observed by Leão et al51.
Load x. deformation for T. domingensis fiber samples reinforced with fiber reinforced single and double layers of waterproofing resin.
From this yield point, the stress–strain curve begins to exhibit a decline in resistance, thereby signaling the degradation of load-bearing support. The inflection point, approximately at 13.5 M, signifies a remarkable shift in stiffness, wherein a knee in the curve demarcates the transition from elastic to plastic behavior52. The post-yield resistance drop may be correlated with the initiation of fiber failure processes, initially through the formation and propagation of microcracks within the material, ultimately leading to fiber rupture. This is characterized by a reduced capacity of the material to bear additional loads, ending in a lessened slope of the stress–strain curve.
In comparison, T. domingensis fibers treated with a double layer present a superior inflection point, at 16.58 M, and those treated with a single layer exhibit an inflection point of 10.25 N. This indicates that the applied treatments might have enhanced the mechanical strength and structural stability of the fibers. Such reinforced behavior could be related to the treatment's effectiveness in shielding the fibers from environmental degradation factors, as well as in fostering improved fiber-matrix adhesion and interaction.
This durability can be attributed to the creation of a superficial coating in the geotextiles by the resin application, reducing the hydromorphic nature of the fiber, making it firmer and resulting in greater durability53. Another benefit obtained by resin coating is resistance to the occurrence of damage caused by granulometric degradation that results from contact with soil particles such as silt and sand54.
For secant stiffness (Jsec), tests of normality distribution of residues showed that treatments T 2 (double layer) [KS = 0.319, p < 0.030; SW = 0.761, p < 0.016] and T3(double layer) [KS = 0.318, p < 0.031; SW = 0.794, p < 0.035] did not show normal distribution. Levene’s test demonstrated that the data did not show homogeneity of variance from time T6(120 days). Mauchly’s sphericity test complied with the sphericity assumption (Mauchly's W = 0.138 c2 (20) = 31.255, p = 0.056). The general result of the ANOVA-MR showed statistically significant differences in tensile strength scores over the proposed degradation time (F (5.478, 1095.73) = 36.896, p < 0.001; η2 = 0.672). In the post hoc longitudinal analyses (Table 2), considering the control samples as the waterproofing resin repetitions for time T1, it was noticed that the secant stiffness decreased after 60 days (T2), with significant differences for all the treatments. In Fig. 6, it was visualized the behavior of the secant stiffness in relation to the treatments along the degradation period.
Secant stiffness (Jsec) of geotextiles over a 180-day period.
From the 120-day exposure to degradation (T5), the untreated geotextile samples demonstrated a better performance compared to the other treatments–even if not that expressive when compared to the effect in the T4 treatment (Average = 6.44%; ΔM = 0.198; p = 1.000, Cohen’s D = 0.087).
This research is distinguished in the field for its innovative incorporation of T. domingensis fibers and environmentally friendly resin to enhance geotextile durability. Our study’s unique approach lies in the precise selection of T. domingensis species widely found in many environments around the world without any other registered study related to geotextile manufacturing. Also the application of waterproofing resins, with a strong emphasis on both ecological compatibility and efficiency seems to be another highlight.
This dual commitment to sustainability and technological innovation in geotextile production sets our work apart in the areas of soil stabilization and slopes erosion control.
The deformation of the geotextile fiber subjected to tension (εii) at times T2 (30 days) [KS = 0.303, p < 0.051; SW = 0.884, p < 0.246] and T 4 (90 days) [KS = 0.319, p < 0.030; SW = 0.821, p < 0.146], as well as treatment with two layers of waterproofing resin at times T4 (90 days) [KS = 0.303, p < 0.037; SW = 0.805, p < 0.246], did not show a normal distribution. Levene's test for tensile deformation (εii) showed that all groups showed homogeneity in variance. Mauchly’s sphericity test did not meet the sphericity assumption (Mauchly’s W = 0.104 C2 (20) = 35.758, p = 0.018).
The overall result of the ANOVA-MR shows that there was a marginally significant difference with low power of effect in tensile deformation scores (εii) over time (F (5, 80,000) = 3.025, p < 0.041; η2 = 0.061). Such results demonstrate a low within-group longitudinal variability for the analyzed treatments. However, in the analysis of variance between treatments longitudinally, the difference was significant with a strong effect (F (10, 80,000) = 291.706, p < 0.004; η2 = 0.714). Figure 7 shows the behavior of the three treatments over a 180-day period in which the geotextile were under degradation.
Tensile deformation (εii) of T. domingensis fiber over a 180-day period for the three waterproofing resin treatments.
Table 3 presents the tensile outcome resulted from the fiber tests. For the deformation data, the treatments with waterproofing resin do not present a significant difference during the field exposure time to degradation. The residual deformation for the samples without waterproofing treatment after a 180-day exposure period was of approximately 21.2%. In the geotextile treated with a layer of waterproofing resin, there was an increase of approximately 9.32%; while for two layers, there was an increase of 24% in deformability.
The obtained data suggests that treatments for both one and two layers of waterproofing resin do not present significant losses in geotextile deformability in comparison with the untreated geotextile—a similar behavior to that noted by Matheus et al55. with manufactured-type geotextiles. This behavior indicates a slower process of structural alteration due to the loss of lignin and alteration of the degree of cellulose crystallinity in the treated samples.
Long-term changes may result in an inverse effect of reducing the values of the mechanical properties of the material if the material is more crystalline, thus, the less empty space will be present and lower the deformability Kakroodi et al56. Plant fibers used in the production of geotextiles have usage potential due to them having a good combination of mechanical and environmental properties57, but due to structural issues, they require associated protective techniques for a good adaptation of technical aspects and resilience in the field.
The geotextile derived from T. domingensis fibers exhibited favorable performance related to its resistance during the period of field exposure caused by climatic variables. The application of a double-layer waterproofing resin has proven effective in delaying fiber degradation for up to 180 days under exposure to climatic variables, excluding temperature58. This extended durability of 180 days aligns well with use in agricultural lands, especially considering the typical crop planting cycles.
This ensures that the geotextile remains effective throughout a complete planting cycle, avoiding interference with mechanized farming processes that could be hindered by more enduring fiber residues in the soil59. Consequently, with appropriate maintenance and treatment, T. domingensis geotextiles can provide a sustainable and advantageous solution in soil bioengineering applications, particularly in agricultural settings.
The T. domingensis in the manufacturing of geotextiles presents itself as an economical alternative to synthetic manufactured geotextiles. Regarding to its availability and the low cost of processing, geotextiles made from T. domingensis offer an affordable solution for soil stabilization, particularly in regions where the plant is abundant. Besides, the potential for treatment using dual crystallization waterproofing technologies emerges as a cost-effective option, enhancing the material's durability, which contributes to the economic viability of this approach60,61.
Regarding carbon emissions, the use of environmentally friendly resins can be part of a broader strategy to reduce the carbon footprint in construction and land stabilization projects. As seen in the study by Ashfaq et al62., the sustainable use of materials in construction projects, including the utilization of industrial by-products like fly ash, can lead to significant savings in costs and carbon emissions. This principle can be extended to the use of T. domingensis fibers. Notably, the amount of resin applied is not enough to cause pollutant damage to the environment. The selection of natural fibers with a low carbon footprint can thus contribute to the overall sustainability of the project, ensuring minimal environmental impact63.
The protective effect of the waterproofing resin has proved the good durability performance of the T. domingensis fibers when exposed to field degradation during a 180-day period, demonstrating that the waterproofing resin was effective in mitigating greater damages resulting from the exposure of geotextiles to degradation; and they did not generate losses already naturally obtained by this fiber.
The applied waterproofing resin creates a coating that covers the fibers, adding extra physical protection and inhibits the degradation of the cellulosic components of the fiber.
The tested natural fiber geotextile presented results close to synthetic geotextiles, with the advantage of waste absence over its uses in the field, which is an element of remarkable importance from the environmental point of view, allowing an improvement in the resistance of geotextiles to natural soil biodegradation.
The research highlights the potential of natural fibers in sustainable uses mostly related as soil bioengineering techniques and highlights the importance of ecological compatibility in their applications. The observed data relies as a reference for future research, opening new avenues for exploring sustainable solutions in soil stabilization and erosion control.
This work not only contributes with valuable information to the field, but also paves the way for innovative and sustainable actions related to the geotextile´s applications.
All data generated or analysed during this study are included in this published article (and its supplementary information files).
Poesen, J. Soil erosion in the anthropocene: Research needs. Earth Surf. Process. Landf. 43, 64–84 (2018).
Gao, Y. F., Hang, L., He, J., Zhang, F. & Van Paassen, L. Pullout behavior of geosynthetic reinforcement in biocemented soils. Geotext. Geomembr. 49, 646–656 (2021).
Holanda, F. S. R. et al. Soil bioengineering in northeastern Brazil: An overview. Rev. Ambi&Agua 16, e2650 (2021).
Mickovski, S. B. & van Beek, L. P. H. Root morphology and effects on soil reinforcement and slope stability of young vetiver (Vetiveria zizanioides) plants grown in semi-arid climate. Plant Soil 324, 43–56 (2009).
Birnbaum, C., Morald, T. K., Tibbett, M., Bennett, R. G. & Standish, R. J. Effect of plant root symbionts on performance of native woody species in competition with an invasive grass in multispecies microcosms. Ecol. Evol. 8, 8652–8664 (2018).
Article PubMed PubMed Central Google Scholar
Wu, Y., Dai, Y., Liu, G., Zeng, J. & Lin, X. Assessment of two whole genome amplification techniques in terms of soil community profiles. Appl. Soil Ecol. 150, 103455 (2020).
Holland, FSR et al. Wave formation and erosion processes on the banks of the Xingó HPP lake. Brazilian Journal of Physical Geography 13, 887–902 (2020).
Rey, F. et al. Soil and water bioengineering: Practice and research needs for reconciling natural hazard control and ecological restoration. Sci. Total Environ. 648, 1210–1218 (2019).
Article ADS CAS PubMed Google Scholar
Basu, G. et al. Bioengineering of river earth embankment using natural fibre-based composite-structured geotextiles. Geotext. Geomembr. 47, 493–501 (2019).
dos Santos Fontes, CS et al. Erosion control with geotextiles from natural fibers on the margin of the São Francisco river. Caminhos de Geografia 22, 25–35 (2021).
Vieira, C. S. & Pereira, P. M. Damage induced by recycled construction and demolition wastes on the short-term tensile behaviour of two geosynthetics. Transp. Geotech. 4, 64–75 (2015).
Sanjana, E. & Mujeeb, M. Properties and importance of geotextiles. Int. J. Adv. Res. Sci. Technol. 12, 25–33 (2022).
Eslamian, M. & Soltani-Kordshuli, F. Development of multiple-droplet drop-casting method for the fabrication of coatings and thin solid films. J. Coat. Technol. Res. 15, 271–280 (2018).
Broda, J. et al. Innovative wool geotextiles designed for erosion protection. J. Ind. Text. 48, 599–611 (2018).
Yan, W. et al. Operational decisions on remanufacturing outsourcing involved with corporate environmental and social responsibility-a sustainable perspective. Sustainability (Switzerland) 10, 1132 (2018).
Rowe, R. K. & Yu, Y. Magnitude and significance of tensile strains in geomembrane landfill liners. Geotext. Geomembr. 47, 439–458 (2019).
Bai, X. et al. Quantitative analysis of microplastics in coastal tidal-flat reclamation in Dongtai China. Front. Environ. Sci. Eng. 16, 1–11 (2022).
Koerner, R. Geotextiles: From Design to Applications (Woodhead Publishing, 2016).
Prambauer, M., Wendeler, C., Weitzenböck, J. & Burgstaller, C. Biodegradable geotextiles–an overview of existing and potential materials. Geotext. Geombr. 47, 48–59 (2019).
Dixon, N., Raja, J., Fowmes, G. & Frost, M. Sustainability Aspects of Using Geotextiles. In Geotextiles 577–596 (Elsevier, 2016). https://doi.org/10.1016/B978-0-08-100221-6.00026-7.
Ok, B., Sarici, T., Demir, A., Talaslioglu, T. & Yildiz, A. Investigation of construction and demolition materials reinforced by geosynthetics. Proc. Inst. Civ. Eng. Eng. Sustain. 176, 285–298 (2023).
Shirazi, M. G. et al. Investigation of tensile strength on alkaline treated and untreated kenaf geotextile under dry and wet conditions. Geotext. Geomembr. 47, 522–529 (2019).
Pott, VJ & Pott, A. Aquatic Plants of the Pantanal Vol. 1 (Embrapa Communication for Technology Transfer, 2000).
dos Santos, MHB, Vieira, IR & De Barros, RFM Ecology, ecosystem services and management of the aquatic plant Typha domingensis pers. Brazilian Journal of Physical Geography 15, 535–545 (2022).
Hadad, H. R., Mufarrege, M. M., Luca, G. A. & Maine, M. A. Long-term study of Cr, Ni, Zn, and P distribution in Typha domingensis growing in a constructed wetland. Environ. Sci. Pollut. Res. 25, 18130–18137 (2018).
Holanda, F. S. R. et al. Geotechnical changes of Thypha domingensis fiber-derived geotextile under degradation due to climatic variables. Int. J. Adv. Manuf. Technol. PREPRINT (2023).
Kew, R. B. G. Plants of the World Online- Typha domingensis Pers (Royal Botanic Gardens, 2023).
Trama, FA et al. The management of Typha domingensis (Typhaceae) affects macroinvertebrate assemblages in the Palo Verde wetland, Guanacaste, Costa Rica. Ecol. Restore. 35, 175–189 (2017).
Feldman, D. Polymer weathering: Photo-oxidation. J. Polym. Environ. 10, 163–173 (2002).
Liu, J. et al. An experimental study on the shear behaviors of polymer-sand composite materials after immersion. Polymers 10, 924 (2018).
Article PubMed PubMed Central Google Scholar
Timothy Stark, B. D., Williamson, T. A., Member, A., Eid, H. T. & Member, S. HDPE geomembrane geotextile interface shear strength. J. Geotech. Eng. 122, 197–203 (1996).
Jeon, H.-Y. Geotextile Composites Having Multiple Functions. In Geotextiles Vol. 1 15–16 (R.M. Koerner, 2016).
Franco, Y. B. et al. Accelerated aging ultraviolet of a PET nonwoven geotextile and thermoanalytical evaluation. Materials 15, 4157 (2022).
Article ADS CAS PubMed PubMed Central Google Scholar
Holland, FSR et al. Degradation of Typha latifolia Linn geotextiles used in soil bioengineering techniques. Forest Science 30, 1147–1160 (2020).
Botvinova, O. A., Panov, Yu. T. & Romanov, S. V. Producing bicomponent sealants based on polyaspartate urea resins. Polym. Sci. Ser. D 13, 407–413 (2020).
Chen, W. et al. New water-based anti-corrosion chemical materials in engineering repairing. Adv. Mat. Res. 287–290, 249–252 (2011).
Bradbury, J. H. & Shaw, D. C. Shrinkproofing of wool with polyglycine from the polymerization of anhydrocarboxyglycine. Text. Res. J. 30, 976–982 (1960).
INMET, IN by M. INMET-National Institute of Meteorology. INMET Preprint at http://www.inmet.gov.br/portal/ (2020).
Martins, M. A., Kiyohara, P. K. & Joekes, I. Scanning electron microscopy study of raw and chemically modified sisal fibers. J. Appl. Polym. Sci. 94, 2333–2340 (2004).
Instron, B. E. C. L. Universal Testing Systems for Tensile Compression and Flexion Tests (INSTRON Brasil equipamentos científicos ltda, 2023).
Cohen, J. Statistical power analysis for the behavioral sciences. Stat. Power Anal. Behav. Sci. https://doi.org/10.4324/9780203771587 (2013).
Haukoos, J. S. & Lewis, R. J. Advanced statistics: Bootstrapping confidence intervals for statistics with “difficult” distributions. Acad. Emerg. Med. 12(4), 360–365 (2005).
Carneiro, J. R., Almeida, P. J. & de Lurdes Lopes, M. Accelerated weathering of polypropylene geotextiles. Sci. Eng. Compos. Mater. 18, 241–245 (2011).
Arthanarieswaran, V. P., Kumaravel, A. & Saravanakumar, S. S. Characterization of new natural cellulosic fiber from Acacia leucophloea bark. Int. J. Polym. Anal. Charact. 20, 367–376 (2015).
Khan, G. M. Thermal characterization of chemically treated coconut husk fibre. Indian J. Fibre Text. Res. 37, 20 (2012).
Huang, C.-C., Yin, S.-C. & Hsu, H.-Y. Comparative study on the pull-out response of nonwoven geotextile in dry and saturated sands. J. GeoEng. 12, 97–107 (2017).
Wang, L., Guo, F. & Wang, S. Prediction model of the collapse of bank slope under the erosion effect of wind-induced wave in the Three Gorges Reservoir Area, China. Environ. Earth Sci. 79, 421 (2020).
Carneiro, J. R., Almeida, P. J. & de Lopes, M. L. Evaluation of the resistance of a polypropylene geotextile against ultraviolet radiation. Microsc. Microanal. 25, 196–202 (2019).
Article ADS CAS PubMed Google Scholar
Abidi, H. et al. Accelerated weathering of textile waste nonwovens used as sustainable agricultural mulching. J. Ind. Text. 50, 1079–1110. https://doi.org/10.1177/1528083719855326 (2019).
Midha, V. K., Joshi, S. & Suresh Kumar, S. Performance of chemically treated jute geotextile in unpaved roads at different in situ conditions. J. Inst. Eng. (India) Ser. E 98, 47–54 (2017).
Leão, A. L. et al. Natural Fibres for Geotextiles. In Handbook of Natural Fibres 280–311 (Elsevier, 2012). https://doi.org/10.1533/9780857095510.2.280.
Mobasher, B. Textile Fiber Composites. In Textile Fibre Composites in Civil Engineering 101–150 (Elsevier, 2016). https://doi.org/10.1016/B978-1-78242-446-8.00006-9.
Sachin Chakravarthy, G., GuhaRay, A. & Kar, A. Experimental investigations on strength and durability of alkali-activated binder-treated natural jute geotextile. Int. J. Geosynth. Gr. Eng. 7(1), 18 (2021).
Liu, S., Wen, K., Amini, F. & Li, L. Investigation of nonwoven geotextiles for full contact flexible mould used in preparation of MICP-treated geomaterial. Int. J. Geosynth. Gr. Eng. 6, 1–12 (2020).
Matheus, E., Palmeira, EM & Agnelli, JAM Degradation of some geosynthetics by ultraviolet radiation and temperature variation. Technical Note. Soils and Rocks 27, 177–188 (2004).
Kakroodi, A. R., Cheng, S., Sain, M. & Asiri, A. Mechanical, thermal, and morphological properties of nanocomposites based on polyvinyl alcohol and cellulose nanofiber from aloevera rind. Res. Artic. Mech. https://doi.org/10.1155/2014/903498 (2014).
Kumar, N. & Das, D. Nonwoven geotextiles from nettle and poly(lactic acid) fibers for slope stabilization using bioengineering approach. Geotext. Geomembr. 46, 206–213 (2018).
Tanasă, F., Nechifor, M., Ignat, M.-E. & Teacă, C.-A. Geotextiles—a versatile tool for environmental sensitive applications in geotechnical engineering. Textiles 2, 189–208 (2022).
Guerreiro Filho, O., Chiba, MK & Ribeiro, RV Geostatistics applied to agricultural and environmental sciences. Bragantia 69, (2010).
Ryu, G.-S., Koh, K.-T., Kim, S.-W. & Kim, D.-G. Development for penetrative performance improving agent to in prevent deterioration of concrete structures. J Korea Concr Inst 17, 489–498 (2005).
Al-Rashed, R. & Jabari, M. Dual-crystallization waterproofing technology for topical treatment of concrete. Case Stud Constr Mater 13, e00408 (2020).
Ashfaq, M., Moghal, A. A. B. & Basha, B. M. The sustainable utilization of coal gangue in geotechnical and geoenvironmental applications. J Hazard Toxic Radioact Waste 26, 03122003 (2022).
Ashfaq, M. & Moghal, A. A. B. Cost and carbon footprint analysis of flyash utilization in earthworks. Int. J. Geosynth. Gr. Eng. 8, 21 (2022).
Thanks to the riverine communities of The Lower São Francisco River, helping in the manufacturing of prototypes of geotextiles based on the experience on the fiber crafts works. Thanks to Dr. Maria Luiza Silveira de Carvalho, professor of Botany at Biology Institute-Universidade Federal da Bahia, Brazil, for the formal identification of the species T. domingensis.
Agronomy Engineering Department, Federal University of Sergipe-UFS, São Cristóvão, Sergipe, Brazil
Francisco Sandro Rodrigues Netherlands, Jeangela Carla Rodrigues De Melo & Gizelio Menezes Boge
Graduate Program in Intellectual Property Science, Universidade Federal de Sergipe-UFS, Avenida Marechal Rondon Jardim S/N - Rosa Elze, São Cristóvão, Sergipe, 49100-000, Brazil
Chemical Engineering Department, Federal University of Sergipe-UFS, São Cristóvão, Sergipe, Brazil
Graduate Program in Materials Science and Engineering (P2CEM), Federal University of Sergipe-UFS, São Cristóvão, Sergipe, Brazil
Chemistry Department, Federal University of Sergipe-UFS, São Cristóvão, Sergipe, Brazil
Marcos Vinícius Quirino dos Santos
Biological Sciences Department, Federal University of Sergipe-UFS, São Cristóvão, Sergipe, Brazil
Marla Ibrahim Uehbe de Oliveira
You can also search for this author in PubMed Google Scholar
You can also search for this author in PubMed Google Scholar
You can also search for this author in PubMed Google Scholar
You can also search for this author in PubMed Google Scholar
You can also search for this author in PubMed Google Scholar
You can also search for this author in PubMed Google Scholar
You can also search for this author in PubMed Google Scholar
You can also search for this author in PubMed Google Scholar
J.M., B.N., G.B. and M.S. conducted experiment and provided data and prepared draft manuscript. F.H., L.S. and E.S. provided the conceptualization, supervision and corrected the manuscript. S.G. helped revise English language. All authors read and approved the final manuscript.
Correspondence to Luiz Diego Vidal Santos.
The authors declare no competing interests.
Springer Nature remains neutral with regard to jurisdictional claims in published maps and institutional affiliations.
Open Access This article is licensed under a Creative Commons Attribution 4.0 International License, which permits use, sharing, adaptation, distribution and reproduction in any medium or format, as long as you give appropriate credit to the original author(s) and the source, provide a link to the Creative Commons licence, and indicate if changes were made. The images or other third party material in this article are included in the article's Creative Commons licence, unless indicated otherwise in a credit line to the material. If material is not included in the article's Creative Commons licence and your intended use is not permitted by statutory regulation or exceeds the permitted use, you will need to obtain permission directly from the copyright holder. To view a copy of this licence, visit http://creativecommons.org/licenses/by/4.0/.
Holanda, F.S.R., Santos, L.D.V., Melo, J.C.R.D. et al. Resistance of the fiber-derived geotextile from Typha domingensis submitted to field degradation. Sci Rep 14, 8648 (2024). https://doi.org/10.1038/s41598-024-56978-3
DOI: https://doi.org/10.1038/s41598-024-56978-3
Anyone you share the following link with will be able to read this content:
Sorry, a shareable link is not currently available for this article.
Provided by the Springer Nature SharedIt content-sharing initiative
By submitting a comment you agree to abide by our Terms and Community Guidelines. If you find something abusive or that does not comply with our terms or guidelines please flag it as inappropriate.
Scientific Reports (Sci Rep) ISSN 2045-2322 (online)
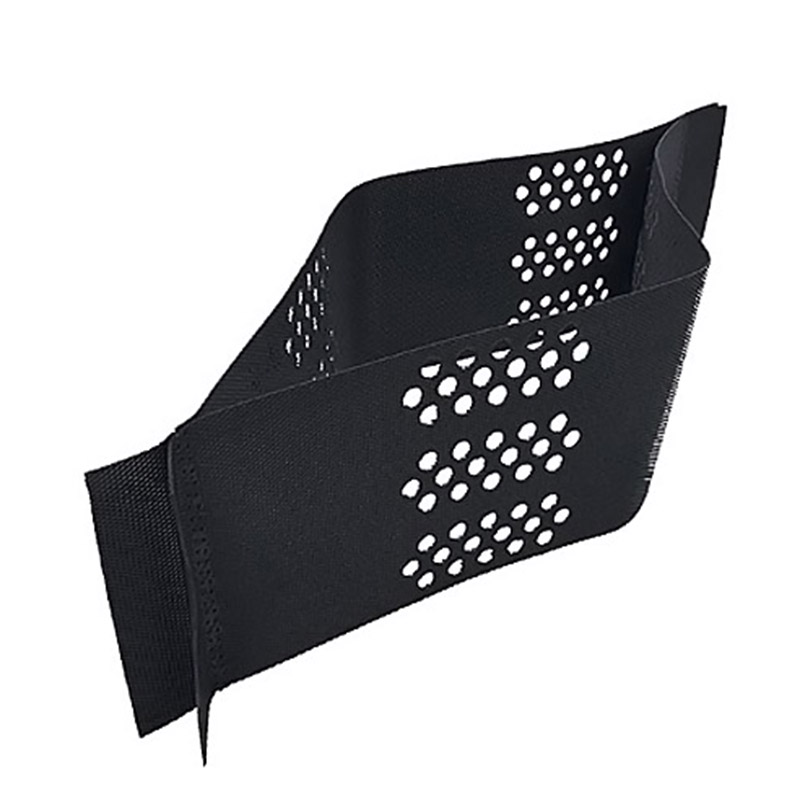
Galvanized Sheet Metal Sign up for the Nature Briefing: Anthropocene newsletter — what matters in anthropocene research, free to your inbox weekly.