In this paper, a one-time digestion method for the determination of arsenic (As), antimony (Sb), selenium (Se), and mercury (Hg) in geological samples was established. The optimum digestion conditions were determined with aqua regia-hydrofluoric acid (HF)-H3BO3 as a complexing open system, and the contents of As, Sb, Se, and Hg in the same digestion solution were determined by chemical vapor generation non-dispersive atomic fluorescence spectrometry (CVG-AFS). For the determination of As, Sb, Se, and Hg, the method detection limits were 0.53 mg/kg, 0.047 mg/kg, 0.009 mg/kg, and 0.00047 mg/kg, respectively, Δ log C was -0.043–0.048, and the %RSD values were lower than 8.5%, performing with good accuracy and precision. This digestion method avoids the fractional processing of each element, simplifies the digestion steps, saves pretreatment time, and improves efficiency. The digestion method also showed wide applicability, high sensitivity, and low detection limits, and it can be used as a quick method for monitoring and controlling heavy metal pollution in large-scale geological samples.
Arsenic (As), antimony (Sb), selenium (Se), and mercury (Hg) are important indicator elements and mandatory items for geochemical sample exploration and geological prospecting (1–5). The elements As and Sb are widely distributed in rocks, soil, and water, and they are toxic and carcinogenic metalloid elements (5–9). Meanwhile, Hg is ubiquitous in various geological media, and its toxicity can affect the central and peripheral nervous systems of humans and animals. As a global environmental pollutant, Hg has attracted much attention (9). As for Se, it is one of the essential trace elements in human body, and a very important antioxidant, which plays an important role in human health. The deficiency and excess of Se is closely related to human health (10). Therefore, accurate analysis of As, Sb, Se, and Hg in geological samples is the basic prerequisite for ecological geochemistry, geochemical sample exploration, and environmental science. Uv Vis Spectrophotometer
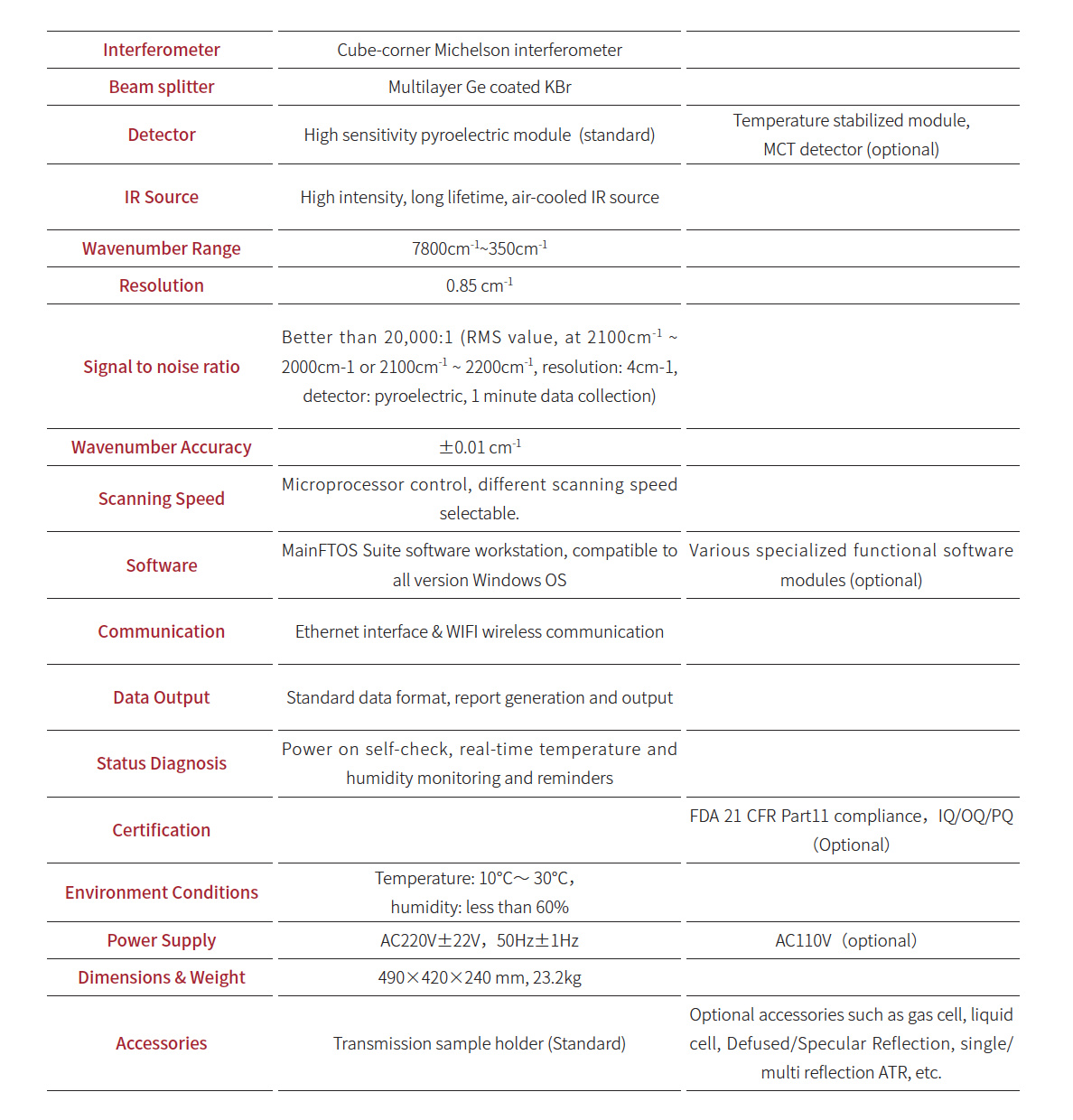
Currently, the methods used to determine As, Sb, Se, and Hg in geological samples include inductively coupled plasma–mass spectrometry (ICP-MS) (11,12), inductively coupled plasma–emission spectrometry (ICP-AES) (13), atomic fluorescence spectrometry (AFS) (14,15), and mercury vapor analysis (MVA). AFS has been widely used to determine As, Sb, Se, and Hg in geological samples because of its advantages of simple operation, high sensitivity, low detection limit, and low operating cost (16,17). However, the pretreatment methods of the four elements are different. As, Sb, and Hg are digested with aqua regia by the water bath, whereas Se is digested with HNO3-HClO4 by heating (18). Different pretreatment methods lead to long analysis times and complicated operations.
The HJ 680-2013 method of the Ministry of Ecology and Environment of China and the EPA-3501 method of the U.S. Environmental Protection Agency (EPA) were used to simultaneously digest As, Sb, Se, and Hg by the microwave-assisted acid dissolution method. However, because of sample weight limitations and the added acid, the method is not suitable for the digestion of all geological samples. After the digestion, the volatile elements are often lost because of transfer and acid removal, and it is difficult to meet the determination of large quantities of geological samples.
Meanwhile, the HCl, HNO3, and HClO4 used in aqua regia water bath digestion, heating digestion, and microwave digestion encounter difficulty destroying the mineral lattice of insoluble samples, which results in the incomplete release of the measured metal elements. In this paper, by adding an appropriate amount of hydrofluoric acid (HF) to the aqua regia system to digest the insoluble minerals, such as silicon and aluminum, while adding H3BO3 to mask the remaining HF, there was no need to remove the acid after digestion. Thus, the procedure saved the acid removed time after the digestion and eliminated the interference with HF. The simultaneous determination of As, Sb, Se, and Hg by one digestion was realized. The method was simple in operation, high in accuracy, had a low detection limit, and was suitable for the determining a large number of geological samples.
Chemical vapor generation non-dispersive atomic fluorescence spectrometry (CVG-AFS) (AFS-V9, Haiguang Instrument Co. Ltd.) was used for the determination of As, Sb, Se, and Hg. Details of the instrument and the operating parameters are summarized in Table I.
Standard solutions used to build the calibration curves were prepared by the appropriate dilution of standard solutions containing 100 mg/L As, Sb, Se and Hg (IGGE). All solutions were prepared using high purity water (18.2 MΩ cm resistivity) obtained from the GN-RO-500 Total Water System (Shuangfeng); HCl, HF, HNO3, H3BO3, thiourea, and ascorbic acid were all analytical grade reagent from Tianjin Chemicals Co. Method validation was carried out using the following reference samples: GSS 5, GSS 7, GSS 8, GSS 16, GSS 20, GSS 24–28 (soil, IGGE), GSD 16, and GSD 19 (stream sediments, IGGE).
To start, 0.5000 g of the sample was added to the polytetrafluoroethylene (PTFE) crucible, with the sample wetted with a small amount of water. Then, 6 mL HCl, 2 mL HNO3, and 2 mL HF were added to the crucible and covered with a watch glass, placed on an electric heating plate at 100 ℃ for 1 h, raised to 150 ℃, and heated for 2 h. After the sample was digested to off-white and cooled to room temperature, 3 mL of the 100 g/L H3BO3 solution was added, diluted to 25 mL with ultrapure water, and shaken well.
To determine As and Sb, 5 mL of supernatant was added to a 10 mL injection tube, and 1 mL HCl and 2 mL 50 g/L of the thiourea-ascorbic acid solution were added, diluted to 10 mL with ultrapure water, shaken well, and placed for 20 min.
To determine Se, 5 mL of supernatant was added to a 10 mL injection tube, and 2 mL HCl was added, diluted to 10 mL with ultrapure water, shaken well, and placed for 20 min.
To determine Hg, 5 mL of supernatant was added to a 10-mL injection tube, and 0.5 mL HCl were added, diluted to 10 mL with ultrapure water, shaken well, and placed for 20 min.
As, Sb, Se, and Hg were determined using CVG-AFS under the same instrument analysis conditions as the standard curve.
Increasing the negative high voltage of the instrument can improve its sensitivity, and increasing the lamp current can increase the fluorescence intensity, but the noise of the instrument will also increase if the negative high voltage and lamp current are too large. According to the concentrations of As, Sb, Se, and Hg, the negative high voltage of 280 V was selected, and the lamp currents of 40, 60, 80, and 30 mA were selected, respectively. Experiments showed that, when the temperature of the atomizer was 200 ℃ and the observation height was 8 mm, the fluorescence intensity of As, Sb, Se, and Hg can be taken into account, and the high fluorescence intensity and low background value can be achieved. Properly reducing the carrier gas flow rate can fully atomize the element hydrides and mercury atoms, and increasing the shielding gas flow rate can effectively reduce fluorescence quenching. When the carrier gas flow rate is 300 mL/min and the shielding gas flow rate is 800 mL/min, the fluorescence intensity of As, Sb, Se, and Hg can be taken into consideration to meet the measurement requirements. Under the same instrument conditions, the acidity of the solution and the concentration of KBH4 are directly related to the fluorescence intensity. Because the content of mercury in the sample is very low, appropriately reducing the concentration of KBH4 can improve the sensitivity of the instrument. Usually, 5% HCl can meet the determination of As, Sb, Se, and Hg. High sensitivity and good reproducibility can be obtained when the concentration of KBH4 is 5 g/L for As and Sb, 20 g/L for Se, and 1 g/L for Hg.
The negative high voltage, lamp current, atomizer height, carrier gas, and shielding gas flow rate were set according to the previously mentioned working conditions, and the As, Sb, Se, and Hg series standard solutions were determined from low to high concentrations. The standard solution series, calibration functions, and correlation coefficient are shown in Table II. The whole process blank solution (n = 12) was prepared according to the prescribed method, and the method detection limits (MDLs) were calculated as the concentration equivalent to three times the standard deviation of blank signal. The detection limits of As, Sb, Se, and Hg were shown in Table II.
As a predeoxidizing agent, thiourea-ascorbic acid can deoxidize As (V) and Sb (V) to As (III) and Sb (III), and the interference of coexisting elements was eliminated or reduced.
The chemical interference of Se determined by CVG-AFS is mainly Cu and Pb. The interference can be eliminated or reduced by adding iron salts, but the condition of eliminating interference is harsh. As shown in Figure 1, 50 g/L of thiourea-ascorbic acid, 38% HCl, 38% HCl-10 g/L Fe3+ solution, and 10 g/L Fe3+ solution were added to eliminate the interference to Se. Reference substances GSD16, GSD19, GSS16, and GSS20 were determined (n = 12). After the addition of thiourea-ascorbic acid, the determination result was significantly lower than the reference value. According to the standard electrode potential value, ΕƟ (deoxidized state of thiourea-ascorbic acid)<ΕƟ(H3AsO4/H3AsO3)<ΕƟ(H3SeO3/Se0), the redox reaction between thiourea-ascorbic acid and H3SeO3 or selenite deoxidize Se (IV) to Se0 leads to low determination results. After HCl-Fe3+ or Fe3+ was added, the determination result of Se significantly deviated from the reference value. The reason may be that Fe3+ reacts with the KBH4 solution to produce Fe2+ before Cu2+, which blocks or slows down the formation of Cu0, thus inhibiting the interference of Cu2+. However, with the increase of Fe3+ content, KBH4 and Fe3+ generate the Fe-B compound to adsorb SeH2, reducing the release efficiency of SeH2. After 38% HCl was added, the determination result was closest to the reference value, indicating that HCl can effectively reduce the interference of coexisting elements, especially the negative interference of Cu2+. The reason may be that the complexation of Cl- increases the oxidation potential of Cu2+, thereby inhibiting the reduction of Cu2+ to Cu0, and preventing the gas-solid phase reaction between Cu0 and SeH2 in the form of collision, adsorption, and wrapping.
FIGURE 1: Reference materials: (a) GSD 16, (b) GSD 19, (c) GSS 16, (d) GSS 20). The determination results (n = 12) of Se in the reference samples (Solid line: certified value; Dotted line: quality control limit line).
Physical interference is the main factor in the determination of Hg with CVG-AFS. Hg is prone to memory effects, and the content in the sample is extremely low, making it difficult to accurately determine. The main error is the residual Hg in the container used for Hg digestion. Residual Hg cannot be completely eliminated by acid soaking, but it can be significantly eliminated by boiling aqua regia.
The soil types are complex, and the parent material, soil formation process, and mineral composition are quite different. Therefore, the digestion efficiency of different soils in the same digestion system is not completely consistent. The addition of HF can rapidly decompose the silicon dioxide, silicate, and insoluble minerals in the sample. The reference samples GSS5, GSS7, and GSS8 were selected for study. Then, 0~5 mL HF was added into the digestion solution, and the remaining HF was removed by heating to determine the optimal dosage of HF.
As shown in Figure 2, the relative errors (%RE) were adopted to evaluate the accuracy (n = 7) of each reference sample. When HF was <0.5 mL, the measured values of As in GSS5 and As and Se in GSS7 are significantly lower than the reference value, indicating that the soil is incompletely digested, and it has little influence on the determination of Sb and Hg. GSS5 is the yellow-red soil in the metal mining area, and GSS7 is the basalt brick red soil, both of which contain insoluble minerals such as gibbsite. HCl and HNO3 are difficult to destroy the mineral crystal lattice, resulting in the incomplete release of metal elements. When the concentration of HF gradually increases, the digestion ability increases, but with the extension of the time to remove the acid by heating, the volatilization loss of As, Sb, Se, and Hg increases significantly, and the measured value decreases significantly. When the weight of the sample was 0.5 g and the amount of HF added was 1.5~2.0 mL, the measurement requirements were met.
FIGURE 2: Reference materials are (a) GSS 5, (b) GSS 7, and (c) GSS8. Reference effect of HF dosage on sample digestion for As, Sb, Se, and Hg measurement (n = 7).
The residual HF in the digestion solution has an adverse effect on the determination and instrument, and the loss of elements will be caused by heating to remove the acid. In this research, H3BO3 was added to mask residual HF to eliminate its adverse effects, and 0~15 mL of 100 g/L H3BO3 solution was added to the digestion solution supplemented with 2 mL HF. As, Sb, Se, and Hg were determined by CVG-AFS according to the “Sample Pretreatment Methods” portion of this paper previously presented. As shown in Figure 3, when 2~5 mL 100 g/L H3BO3 solution was added, the measured values of As, Sb, Se, and Hg were satisfactory. When H3BO3 was insufficient (<2 mL), HF cannot be fully masked, resulting in high results of As, Sb, Se, and Hg; When H3BO3 was added in excess (>5 mL), the generated flocculent precipitate affected the combustion of the atomizer, resulting in higher results and larger deviations. In summary, 3~4 mL 100 g/L H3BO3 solution was added as masking agent.
FIGURE 3: Reference materials: (a) GSS 5, (b) GSS 7. Effect of H3BO3 dosage on determination of As, Sb, Se, and Hg measurement (n = 7).
According to the specified method, the reference samples GSS24–28 were measured. For each analyzed reference sample, the average determined value (n = 7), certified value, accuracy results (Δ log C) and relative standard deviation (%RSD) (n = 7) were collected in Table Ⅲ.
Results accuracy was evaluated by the quality test proposed by the International Geological Correlation Programme (IGCP) comparing the differences between obtained and recommended values by the expression of Δnlog C ( log Cs–log Ci), where Ci and Cs are the certified and measured value, respectively. According to IGCP criteria, Δ log C of the reference samples analyzed in this work would be between -0.05 and 0.05 for As, Sb, Se, and Hg. The results in Table III showed that Δ log C of As, Sb, Se, and Hg determination were -0.043–0.048, showing good accuracy. The %RSD values of As, Sb, Se, and Hg determination were lower than 8.5%, which showed good precision. Therefore, the measurement method developed in this work using CVG-AFS analysis proved to be a valid method for determining As, Sb, Se, and Hg in geological samples.
In this work, a one-time digestion method for the determination of As, Sb, Se, and Hg in geological samples was established. The optimum digestion conditions were determined with aqua regia-HF-H3BO3 as the complexing open system, and the contents of As, Sb, Se, and Hg in the same digestion solution were determined by CVG-AFS. This digestion method has wide applicability, high sensitivity, good accuracy and precision, low detection limit, and it can be used as a quick method for monitoring and controlling heavy metal pollution in large-scale geological samples.
The authors acknowledge financial support from the Project of China Geological Survey (Grant Number DD20208069).
The authors have no conflict of interest.
(1) Zhu, Y.; Williams, P. N.; Meharg, A. A. Exposure to Inorganic Arsenic from Rice: A Global Health Issue? Environ. Pollut. 2008, 154, 169–171 (2008). DOI: 10.1016/j.envpol.2008.03.015
(2) Meharg, A. A.; Williams, P. N.; Adomako, E. et al. Geographical Variation in Total and Inorganic Arsenic Content of Polished (White) Rice. J. Environ. Sci. Technol. 2009, 43, 1612–1617. DOI: 10.1021/es802612a
(3) Rintala, E.; Ekholm, P.; Koivisto, P.; Peltonen, K.; Venalainen, E. The Intake of Inorganic Arsenic from Long Grain Rice and Rice-Based Baby Food in Finland – Low Safety Margin Warrants Follow Up. Food Chem. 2014, 150, 199–205. DOI: 10.1016/j.foodchem.2013.10.155
(4) Carbonell-Barrachina, A. A.; Ramirez-Gandolfo, A. et al. Essential and Toxic Elements in Infant Foods from Spain, UK, China and USA. J. Environ. Monit. 2012, 14, 2447–2455. DOI: 10.1039/C2EM30379E
(5) Chen, G.; Chen, T. SPE Speciation of Inorganic Arsenic in Rice Followed by Hydride-generation Atomic Fluorescence Spectrometric Quantification. Talanta 2014, 119, 202–206. DOI: 10.1016/j.talanta.2013.11.016
(6) Watts, M. J.; Reilly, J.; Marcilla, A. L.; Shaw, R. A.; Ward, N. I. Field Based Speciation of Arsenic in UK and Argentinean Water Samples. Environ. Geochem. Health 2010, 32, 479–490 (2010). DOI: 10.1007/s10653-010-9321-y
(7) Gebel, T. W. Genotoxicity of Arsencial Compounds. Int. J. Hyg. Environ. Health 2001, 203, 249–262 (2001). DOI: 10.1078/S1438-4639(04)70036-X
(8) Sundar, S.; Chakravarty, J. Antimony Toxicity. Int. J. Environ. Res. Public Health 2010, 7, 4267–4277. DOI: 10.3390/ijerph7124267
(9) Jagtap, R.; Krikowa, F.; Maher, W.; Foster, S.; Ellwood, M. Jagtap, R.; Krikowa, F.; Maher, W.; Foster, S.; Ellwood, M. Measurement of Methyl Mercury (I) and Mercury (II) in Fish Tissues andSediments by HPLC-ICPMS and HPLC-HGAAS. Talanta 85, 49–55. DOI: 10.1016/j.talanta.2011.03.022
(10) Sathrugnan, K.; Hirata, S. Determination of Inorganic Oxyanions of As and Se by HPLC–ICPMS. Talanta 2004, 64, 237–243. DOI: 10.1016/j.talanta.2004.02.016
(11) Romanovskiy, K. A.; Bolshov, M. A.; Münz, A. V.; Temerdashev, Z. A.; Burylin, M. Y.; Sirota, K. A. A Novel Photochemical Vapor Generator for ICP-MS Determination of As, Bi, Hg, Sb, Se and Te. Talanta 2018, 187, 370–378. DOI: 10.1016/j.talanta.2018.05.052
(12) Vieira, M. A.; Ribeiro, A. S.; Curtius, A. J. Determination of As, Ge, Hg, Pb, Sb, Se and Sn in Coal Slurries by CVG-ETV-ICP-MS Using External or Isotopic Dilution Calibration. Microchem. J. 2006, 82, 127–136. DOI: 10.1016/j.microc.2006.01.002
(13) Welna, M.; Szymczycha-Madeja, A. Effect of Sample Preparation Procedure for the Determination of As, Sb and Se in Fruit Juices by HG-ICP-OES. Food Chem. 2014, 159, 414–419. DOI: 10.1016/j.foodchem.2014.03.046
(14) Chen; Y.-W.; Zhou, M.-D.; Tong, J.; Belzile, N. Application of Photochemical Reactions of Se in Natural Waters by Hydride Generation Atomic Fluorescence Spectrometry. Anal. Chim. Acta 2010, 671, 9–26. DOI: 10.1016/j.aca.2005.02.079
(15) Chen, B.; Krachler, M.; Gonzalez, Z.; Shotyk, W. J. Improved Determination of Arsenic in Environmental and Geological Specimens Using HG-AFS. Anal. Atom. Spectrom. 2005, 20, 95–102. DOI: 10.1039/b416142d
(16) Cava-Montesinos, P.; Cervera, M. L.; Pastor, A.; Guardia, M. Determination of As, Sb, Se, Te and Bi in Milk by Slurry Sampling Hydride Generation Atomic Fluorescence Spectrometry. Talanta 2004, 62, 173–182. DOI: 10.1016/S0039-9140(03)00411-9
(17) de Santana, F. A..; Portugal, L. A.; Serra, A. M.; Ferrer, L.; Cerdà, V.; Ferreira, S. Development of a MSFIA System for Sequential Determination of Antimony, Arsenic and Selenium Using Hydride Generation Atomic Fluorescence Spectrometry. Talanta 2016, 156, 29–33. DOI: 10.1016/j.talanta.2016.04.063
(18) García, J. B.; Krachler, M.; Chen, B.; Shotyk, W. Improved Determination of Selenium in Plant and Peat Samples Using Hydride Generation-Atomic Fluorescence Spectrometry (HG-AFS). Anal. Chim. Acta 2005, 534, 255–261. DOI: 10.1016/j.aca.2004.11.043
Wen-Zhi Zhao, Bing-Bing Zhang, Yuan Zhang, and Bing Lu are with the Center for Harbin Natural Resources Comprehensive Survey at the China Geological Survey, in Harbin, China. Jiu-Fen Liu is with the Command Center for Natural Resources Comprehensive Survey at the China Geological Survey, in Beijing, China; Direct correspondence to Jiu-Fen Liu at liujiufen123@163.com●
A Brief Review of the Latest Spectroscopic Research in Environmental Analysis
Spectroscopic analytical techniques are crucial for the analysis of environmental samples. This review emphasizes the latest advancements in several key spectroscopic methods, including atomic, vibrational, molecular, electronic, and X-ray techniques. The applications of these analytical methods in detecting contaminants and other environmental applications are thoroughly discussed.
Tracking Molecular Transport in Chromatographic Particles with Single-Molecule Fluorescence Imaging
An interview with Justin Cooper, winner of a 2011 FACSS Innovation Award. Part of a new podcast series presented in collaboration with the Federation of Analytical Chemistry and Spectroscopy Societies (FACSS), in connection with SciX 2012 ? the Great Scientific Exchange, the North American conference (39th Annual) of FACSS.
Analyzing Soil Acidification Using Mid-Infrared Spectroscopy
A recent case study in southern Australia shows how mid-infrared (MIR) spectroscopy can analyze overall soil quality by measuring its acidity.
Advanced Raman Techniques for Micro- and Nanoplastics Detection
A recent review article discusses four advanced Raman techniques, including surface-enhanced Raman scattering (SERS), Raman tweezers (RTs), tip-enhanced Raman scattering (TERS), and Raman mapping/imaging (RM/RI), and how these techniques are being applied for the detection of micro- and nanoplastics.
Tröger’s Base Derivatives Studied Using HPLC
Scientists from the Czech Republic recently created new practices to study different Tröger’s base isomers.
New LiDAR System Tested to Further Automated Recognition Systems
Korean scientists recently tested a new light detection and ranging (LiDAR)-based system for improving autonomous recognition systems.
A Brief Review of the Latest Spectroscopic Research in Environmental Analysis
Spectroscopic analytical techniques are crucial for the analysis of environmental samples. This review emphasizes the latest advancements in several key spectroscopic methods, including atomic, vibrational, molecular, electronic, and X-ray techniques. The applications of these analytical methods in detecting contaminants and other environmental applications are thoroughly discussed.
Tracking Molecular Transport in Chromatographic Particles with Single-Molecule Fluorescence Imaging
An interview with Justin Cooper, winner of a 2011 FACSS Innovation Award. Part of a new podcast series presented in collaboration with the Federation of Analytical Chemistry and Spectroscopy Societies (FACSS), in connection with SciX 2012 ? the Great Scientific Exchange, the North American conference (39th Annual) of FACSS.
Analyzing Soil Acidification Using Mid-Infrared Spectroscopy
A recent case study in southern Australia shows how mid-infrared (MIR) spectroscopy can analyze overall soil quality by measuring its acidity.
Advanced Raman Techniques for Micro- and Nanoplastics Detection
A recent review article discusses four advanced Raman techniques, including surface-enhanced Raman scattering (SERS), Raman tweezers (RTs), tip-enhanced Raman scattering (TERS), and Raman mapping/imaging (RM/RI), and how these techniques are being applied for the detection of micro- and nanoplastics.
Tröger’s Base Derivatives Studied Using HPLC
Scientists from the Czech Republic recently created new practices to study different Tröger’s base isomers.
New LiDAR System Tested to Further Automated Recognition Systems
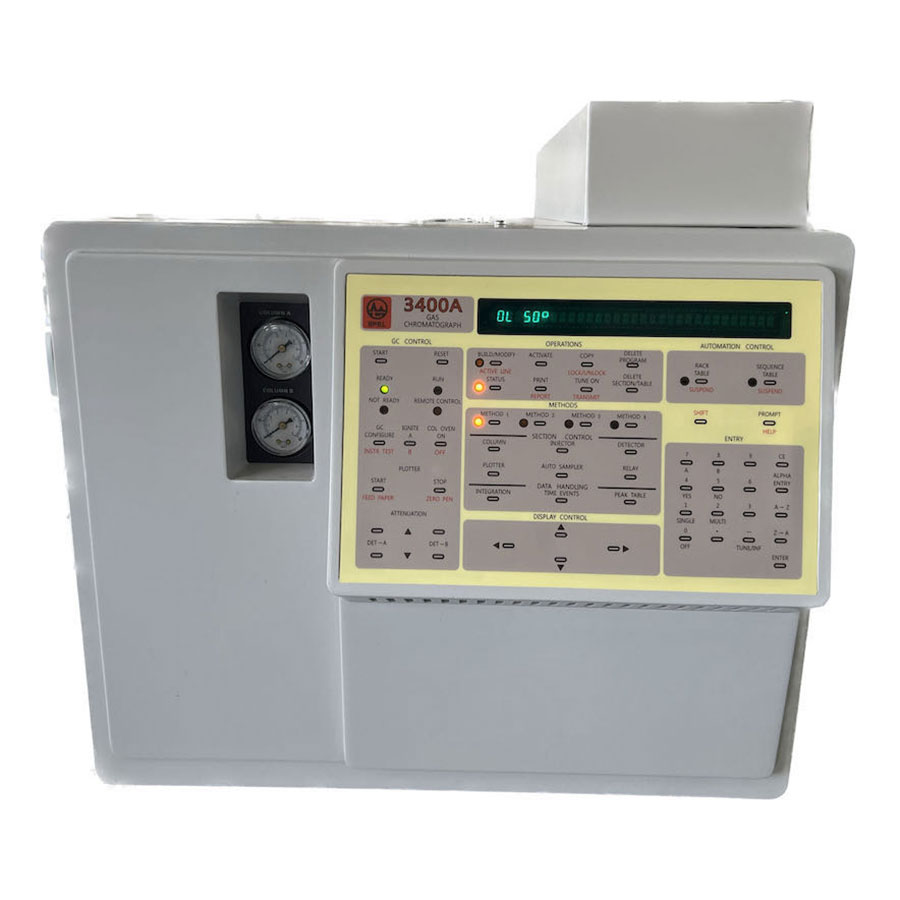
Fourier Infrared Spectroscopy Korean scientists recently tested a new light detection and ranging (LiDAR)-based system for improving autonomous recognition systems.