Thank you for visiting nature.com. You are using a browser version with limited support for CSS. To obtain the best experience, we recommend you use a more up to date browser (or turn off compatibility mode in Internet Explorer). In the meantime, to ensure continued support, we are displaying the site without styles and JavaScript.
Scientific Reports volume 13, Article number: 16123 (2023 ) Cite this article Ammonium Sulphate Price Per Kg
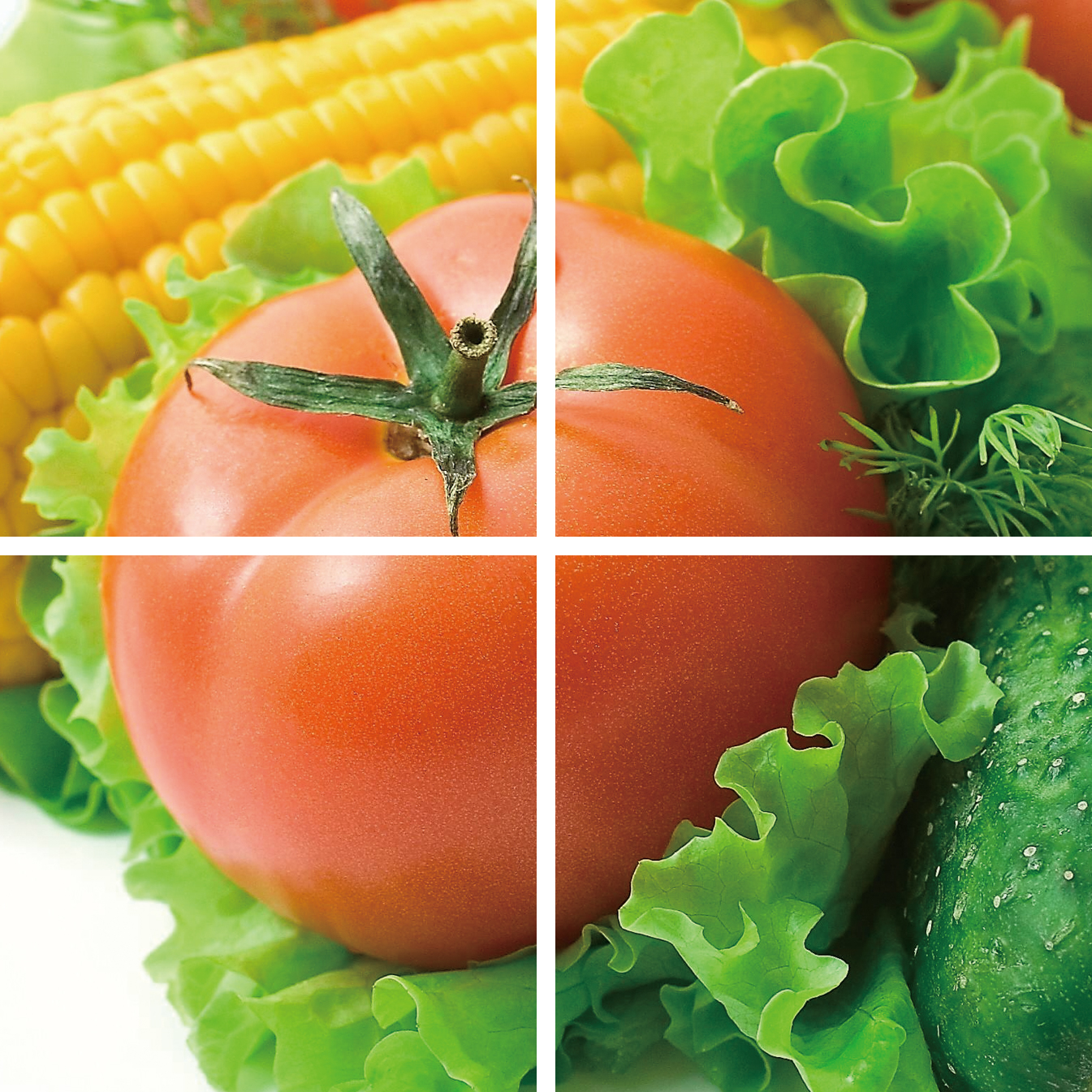
Sarcocystis spp. infects water buffaloes (Bubalus bubalis) causing sarcocystosis. In the present study, Sarcocystis fusiformis was recognized in Egyptian water buffaloes based on histological observation and molecular analysis of internal transcribed spacer 1 (ITS1), 18S ribosomal RNA (18S rRNA) and cytochrome c oxidase subunit I (COX-1) gene fragments. Chemotherapy and vaccines against Sarcocystis spp. could potentially target proteases because they may play a crucial role in the infection. Cysteine proteases are multifunctional enzymes involved in vital metabolic processes. However, the involvement of proteases in S. fusiform infection has not yet been characterized. Here, the purification and study on some biochemical properties of protease isolated from cysts of S. fusiform were carried out. Protease with a molecular weight of 100 kDa was purified. LC–MS/MS analyzed the protein sequence of purified protease and the data suggested that the enzyme might be related to the cysteine protease. The purified protease exhibited maximum activity at pH 6 and a temperature of 50 °C. The Michaelis–Menten constant (Km), the maximum velocity (Vmax), and the turnover number (Kcat) were determined. The complete inhibition effect of cysteine inhibitors indicated that the purified enzyme is a cysteine protease. The results suggested that S. fusiform proteolytic enzyme may be necessary for parasite survival in water buffaloes by digesting host tissues. Therefore, cysteine protease could be a suitable target for vaccinations.
Muscular sarcocystosis is an Infectious disease caused by Apicomplexan protozoa of the genus Sarcocystis spp. The life cycle of this genus involves two hosts including an intermediate host (herbivorous prey: asexual stage and cyst-forming in muscle) and the definitive host (carnivorous predator: intestinal sexual phase and mature oocysts development)1. More than 200 species of Sarcocystis have been identified2, and they infect humans and a variety of domestic like cattle, buffaloes, sheep, pigs, and wildlife animals globally, leading to serious health and financial damages3,4. Sarcocystis-infected animals suffer from weight loss, low milk yield, anemia, miscarriage, and even death from severe infections5. Chronic sarcocystosis may lead to financial issues because of lessened meat, milk, and wool6 and the impact on human health due to the consumption of infected raw meat5. Sarcocystis spp. infection can result in mortality7. There are two types of Sarcocystis cysts, microscopic and macroscopic. The cysts are detected in the tongue, esophagus, diaphragm, heart, and skeletal muscles in the infected-Sarcocystis ruminants8,9. There are four species of Sarcocystis infecting water buffalo (B. bubalis) as intermediate hosts; S. fusiformis and S. buffalonis, S. levinei, and S. dubeyi. Cats are the definitive host for S. fusiformis and S. buffalonis, dogs are the definitive host for S. levinei, while S. dubeyi has not yet been identified10.
The overall prevalence rate of Sarcocystis infection in the examined slaughtered buffaloes at Egyptian abattoirs varies between 20 to 86% depending on hosts triated muscles, age, gender, geographic location/final host (cat and dog), size of cyst (microscopic and macroscopic), species and diagnostic method9,11,12,13,14. The high prevalence of Sarcocystosis in water buffaloes in Egypt is considered to be a genuine issue for both general health and the animal economy.
Parasitic enzymes which play an important role in host–parasite interactions and in the disease process, are attractive targets for diagnostic assay and vaccine development15. Proteases catalyze the hydrolysis of peptide bonds and they play important role in the development and maintenance of parasitic infections. Eukaryotic proteases can be classified into four catalytic classes: serine (e.g., trypsin, chymotrypsin, elastase), cysteine (thiol) (e.g., rennin, cathepsin) aspartic (e.g., pepsin) and metalloprotease (e.g., carboxypeptidase, thermolysin)16,17. Proteases have multiple functions for instance enable host invasion, tissue digestion and destruction, immune system evasion, clot dissociation and enhanced vascular permeability. Understanding the structural or functional relevance and substrate specificity of proteases can provide a basis for the discovery of new lead for antiparasitic therapy18,19,20,21.
The aim of the present work is to identify species of Sarcocystis spp. from Egyptian water buffaloes based on histological observation and molecular analysis. The purification and characterization of cysteine protease from identified Sarcocystis fusiformis is the second goal.
Experimental procedures were carried out in compliance with relevant guidelines. This study was approved by Medical Research Ethics Committee of National Research Centre, Cairo, Egypt (Subject N0. 1-3-1-5). The study is reported in accordance with ARRIVE guidelines (https://arriveguidelines.org).
Sarcocystis infected samples from the esophageal muscles of nineteen water buffalo (male, 3–5 years) were collected during slaughtering at Bassatin abattoir, Egypt, after being identified by the specialized veterinary doctor during the period from January 2021 to January 2022. Specimens containing macroscopic, sarcocysts were isolated directly. Morphological details were carried out by the naked eye and cysts were teased out by a fine forceps, washed with saline, and stored at − 20 °C for enzymes and DNA extractions.
The infected muscles were formalin-buffered at 10%, dehydrated and paraffin-embedded. Hematoxylin (H) and eosin (E) were used to stain the muscle sections (5 μm). An Olympus CX 51 light-microscope was used to examine the stained muscles, and a C100-camera was used to take photomicrographs.
Genomic DNA (gDNA) extraction was done using Thermo Scientific Gene JET kit, according to the manufacturer’s tissue protocol.
The identification of Sarcocystis spp. was performed through PCR targeting the 18S ribosomal RNA (rRNA), mtDNA cyclo-oxygenase 1 (COX1) genes and Internal transcribed spacer1 (ITS1) rDNA gene region. We used pair primers of 18S ribosomal RNA (rRNA) (SF1/SR1) that are common for the genus Sarcocystis as previously described22 (Table 1). Primers for ITS-1 and COX1 genes were designed from the conserved regions in S. fusiformis, S. buffalonis, S. levinei and S. sinensis as detected by the alignment using GENtle software. The PCR reaction was conducted in a 50 µl-volume, containing 1 × Thermo Scientific Phusion HF buffer (1.5 mM MgCl2), 0.5 µM each primer, 50–150 ng DNA template, 1-unit Thermo Scientific Phusion DNA Polymerase and 200 µM dNTP in final reaction concentration. The thermal protocol of PCR was as follows: initial denaturation at 98 °C for 30 s, 30 cycles at 98 °C for-10 s, annealing at 60 °C for-30 s, and 72 °C for-30 s then a final extension step for-10 min at 72 °C. PCR amplicons were visualized by agarose gel electrophoresis (1.5%) with ethidium bromide. The DNA amplicons were purified from the agarose gels using Thermo Scientific GeneJET kit, Nanodrop spectrophotometric readings were used to quantify the results according to the manufacturer's recommendations. Sanger sequencing for the amplicons was performed by Macrogen (Seoul, South Korea) using the same primers as for PCR. The sequencing for 18SrRNA amplicons were done by new set specific for S. fusiformis (SF2/SR2) (Table 1).
The protease activity was assessed by employing the Lemos et al. method23 and azocasein as a nonspecific protease substrate. A reaction mixture (1 ml) containing 0.1% azocasein was mixed with a 20 mM Tris–HCl buffer solution with a pH of 7.2, and the mixture was then incubated at 37 °C for 1h. After incubation, 100 µl of TCA (20%) was added to stop the reaction, the mixture was then centrifuged at 12,000g for 5-min to remove the precipitate. An absorbance change of 0.1 at 366 nm is equivalent to one unit per hour.
On the basis of the generation of free amino groups, the affinity of the isolated enzyme towards several native protease-substrates including casein, albumin, gelatin, collagen type I, hemoglobin, and fibrin was examined. The isolated enzyme (10U), 5 mg of each protease-substrate, and 50 mM Tris–HCl buffer, pH 6.0 were incubated at 50 °C. After one hour incubation, 100 µl of TCA (20%) was added to stop the reaction, the mixture was then centrifuged at 12,000g for 5-min to remove the precipitate. Following that, 100 µl of ninhydrin reagent and 0.5 ml of the reaction mixture were incubated at 84 °C for 5-min24. After cooling, absorbance at 570 was recorded. Isoleucine was employed as a standard, and the amount of amino acid liberated in µg/h was equivalent to one unit.
The assay for cysteine protease was conducted using Arnon25 method. N-benzoyl-arginine-p-nitroanilide–HCl (BAPNA-HCl), a specific cysteine protease, was dispersed in 10 mM dimethyl-sulfoxide. One ml of the reaction mixture contains 100 µl of enzyme mixed with 800 µl of 50 mM sodium phosphate buffer, pH 6.0, containing 5 mM mercaptoethanol. The reaction started by adding 100 µl of 1 mM substrate followed by incubation of the mixture at 50 °C for 1 h. The cysteine protease activity was measured at 405 nm. One-Unit proteolytic activity defines as the amount of enzyme generating 1 µl mol of p-nitroaniline/h.
By employing the Bradford method, protein content was measured26.
The 400 mg of cysts were blended with a 50 mM Tris–HCl buffer (pH 7.0) and centrifuged in a cooled centrifuge for 15-min at 12,000 rpm. The supernatant was designed as crude extract and maintained at − 20 °C for subsequent examination.
In a cooling water bath, solid ammonium-sulfate was gently added to the crude extract until obtained 80% saturation. After centrifugation for 20-min at 12,000 rpm and 4 °C, the produced precipitate was dissolved and dialyzed against 50 mM Tris–HCl buffer, pH 7.0 at 4 °C overnight and centrifuged for 20-min at 12,000 rpm and 4 °C. The produced supernatant was designed as ammonium-sulphate fraction.
The DEAE-Sepharose column (15 × 1.6 cm) was equilibrated by 50 mM Tris–HCl buffer, pH 7.0 and then the ammonium-sulfate fraction was applied to it. At a flow rate of 60 ml/h, the enzyme was eluted utilizing a stepwise gradient from 0.0 to 0.5 M NaCl-in the same buffer, and fractions of 3 ml were compiled. The caseinolytic activity in the collected fractions was assessed, and the active peaks were pooled and stored at − 20 °C for further studies.
The sodium dodecyl sulfate–polyacrylamide gel electrophoresis (SDS-PAGE) technique27 was utilized to assess the molecular mass and homogeneity of the obtained enzyme.
The protein sequence analysis of protease from S. fusiformis was performed nano-Reverse Phase LC coupled to a QExactive Hybrid Quadrupole—Orbitrap mass spectrometer (Thermo Scientific, Bremen, Germany) through a nanoelectrospray ion source (ThermoScientific, Bremen, Germany) as following: a protein band of protease was in gel-digested for 3 h at 37 °C by trypsin. The resulting peptide mixture was resolved and applied on LC–MS/MS. Raw MS files were analyzed by the MaxQuant v1.5.3.3 proteomics software package. Precursor and MS/MS mass tolerance was set to 20 ppm for the first search (for the identification of the maximum number of peptides for mass and retention time calibration) and 4.5 ppm for the main search (for the refinement of the identifications). Protein and peptide false discovery rate (FDR) were set to 1%. FDR was calculated based on the number of spectra matched to peptides of a random proteome database (reversed sequence database) in relation to the number of spectra matching to the reference proteome. Peptide features were aligned between different runs and masses were matched (“match between runs” feature), with a match time window of 3 min and a mass alignment window of 20 min. Protein quantification was performed using the iBAQ algorithm through MaxQuant software.
The characterization of the isolated enzyme was evaluated using the caseinolytic standard assay conditions as mentioned above23. The optimal pH was studied by applying 50 mM sodium acetate and Tris–HCl buffers with pH ranges of 5.0–6.0 and 7.0–9.0, respectively, for 1 h. The optimal temperature was studied by allowing the reaction mixture to be incubated at various temperatures ranging (25–70 °C) for 1 h. The impact of temperature on the stability of the enzyme was investigated. Before substrate addition, the enzyme was pre-incubated for 30-min at various temperatures ranging (25–70 °C), then cooled in an ice bath, and the residual activity was identified.
The steady-state kinetic parameters of the isolated enzyme were studied at pH 6.0 and 50 °C with a range of azocasein concentration of 0.025 to 0.20 mg/ml, and incubation for 1h under the standard caseinolytic assay conditions. Michaelis–Menten plot using nonlinear regression analysis of the Graph-Pad prism program version 5 has been employed to determine the Km, and Vmax values.
Before adding substrate, the enzyme was individually pre-incubated for 30 min at 37 °C with metal ions at 2 and 5-mM final concentrations. For protease inhibitors, the reaction mixture included the BAPNA-HCl a specific cysteine substrate and inhibitor at 2 and 5-mM. The residual activity was then measured by the method of Arnon25. In the absence of an inhibitor or metal ion, proteolytic activity was considered to be 100%.
All experimental procedures were carried out in compliance with relevant guidelines.
The sarcocystis-infected samples from the esophagus were collected separately from 19 water buffaloes slaughtered at the El-Basaten abattoir in Cairo. All infected tissues collected from water buffaloes exhibited macroscopic cysts (Fig. 1A,B). The macroscopic cysts were milky white and opaque in color, with a fusiform shape and ranged in size from 5–15 mm long × 1–4 mm wide (n = 22). Hematoxylin and eosin staining for this macroscopic cyst is shown in Fig. 1C–F. The examination of the histological section has shown that the cyst was bordered by a smooth thin cyst wall and the cyst cavity was divided into typical compartments of different sizes by thin septa. Two parasitic stages were within the cysts, metrocytes that acquired a pale stain near the margin of the cyst and the slender curved bradyzoites which filled most of the cyst, tightly packed in the interior area of the cyst and showed a dark stain.
Macroscopical: oesophagus from water buffalo heavily infected with Sarcocystis fusiformis. The infection appeared as macroscopic spindle-shaped and milky white sarcocysts embedded in the esophagus muscles (A,B). Histological: Section of sarcocyst stained with hematoxylin and eosin (C, × 40; D,E × 100; F × 400). Thin sarcocyst wall (opposing arrowheads), septa (S) groups of bradyzoites (br), and faint staining metrocytes (me).
The three selected genes (18S rRNA, ITS1, and mitochondrial COX1) were successfully amplified and sequenced from 15 isolates of sarcocystis. A BLAST comparative analysis of 18S rRNA gene amplicons with 1.8 kbp revealed that the isolates related to Sarcocystis fusiformis reference sequences with identity ranged from 89 to 97%. As the PCR products of 18S rRNA were amplified by a common primer for Sarcocystis spp., PCR amplification with specific primers for S. fusiformis was carried out, yielding PCR products of 553 bp for more conformation. The alignment of these partial 18S rDNA nucleotide sequences with that from others deposited in GenBank showed similarity ranging from 90 to 99% to S. fusiformis. The phylogenetic tree was generated based on the sequences with high identity (Fig. 2A). Our isolates are closely related to sequences from Egypt and China. The 700 bp-long ITS1 sequences of isolates showed 87–96% similarity to S. fusiformis in the GenBank, whereas the comparison of the 278 bp-long COX1 sequences of sarcocystis isolates exhibited 98–99% similarity to S. fusiformis sequences. Phylogenic trees based on ITS1 and COX1 were generated using some species with sequences having high percentages of identity (Fig. 2B,C). The later trees showed a high similarity to Indian and Egyptian isolates of S. fusiformis. A phylogenetic tree representing fifteen clones shows 48 clones of S. fusiformis and eight other closely related species (three S. hirsuta, four S. buffalonis, and one S. cafferi) (Fig. 3).
A phylogenetic tree for Sarcocystis spp. generated by a Fast Tree analysis using an NCBI alignment of (A) 18SrRNA, ITS1 (B), and COX1 (C) nucleotides. (*) Sarcocystis isolate.
A phylogenetic tree form Sarcocystis spp. from water buffaloes generated by a FastTree analysis using an NCBI alignment of18SrRNA showing 50 clones.
The purification scheme of protease from S. fusiformis cysts is summarized in Table 2. Two steps were used to purify the enzyme. The initial step of purification is 80% ammonium sulfate precipitation. The dialyzed enzyme that was obtained from the ammonium sulfate process was loaded onto the DEAE-Sepharose column. When Tris–HCl buffer with varying concentrations of NaCl (0.0–0.5 M) was used as the elution buffer on DEAE-Sepharose, six peaks of enzyme activity were separated (Fig. 4). Separate peak-forming fractions were pooled, concentrated, and measured for protease activity. The 0.2 M NaCl eluted peak showed 20-fold purification and retained the major protease activity (as shown in Table 2). SDS-PAGE was used to verify the preparation's purity, and the peak representing protease protein migrated as a single band with a molecular weight of about 100 kDa, as shown in Fig. 5.
A typical chromatography profile of ammonium sulfate fraction of Sarcocystis spp. protease from infected water buffaloes on DEAE-Sepharose (15 × 1.6 cm) pre-equilibrated with 50 mM Tris–HCl buffer, pH 7.0. Three ml fractions were collected at a flow rate of 60 ml/h and 4 °C.
SDS-PAGE for homogeneity and molecular weight determination of protease from Sarcocystis fusiformis. (M) Protein markers; (1) Crude extract, (2) Ammonium sulfate fraction, (3) Purified enzyme of 0.2 M NaCl DEAE-Sepharose fraction.
SDS-PAGE protein band of protease from S. fusiformis was cut, tryptic digested and LC–MS/MS analyzed. The identified peptide fragments (Table 3), with posterior error probability score < 1% and protein/peptide false discovery rate < 1%, were aligned against Toxoplasma sp. proteases. Five peptide fragments out of 18 identified peptide fragments are highly similar to the N- and C-terminal ends of Toxoplasma sp. Cysteine protease and ovarian tumor unit domain-containing cysteine proteases. Out of these peptides 3 long peptides (ALVGVSASPKSGQVTSPFFVPLVGSPPYLSAYGEVETPAR, AVVASASSTSAFRLTGSAGVPFSDAASGGYVKTQDVSR and LGLAPGECWR) were homologous to a group of 16 OTU-like cysteine protease domain-containing proteins (group 1) of MW ~ 143 kDa and accession numbers of: A0A0F7USW2; S8GF61; S7UED7; A0A7J6K940; A0A425HSH9; A0A2G8Y620; A0A151HS29; A0A139XQK1; A0A125YX03; A0A086QNK3; A0A086PWS5; A0A086LU92; A0A086L465; A0A086JQ18; A0A086JLS6 and A0A2T6IPI8 (Fig. S1A). Similarly, one peptide (VDAAELDLDR) was homology to a second group of 22 OTU-like cysteine protease domain-containing proteins (group 2) of MW ~ 24 kDa and accession numbers of: A0A7J6KF34; A0A125YIL; S7VTI5; A0A125YIF9; A0A086PG20; V4YYX2; A0A086JH25; A0A086LJ08; S8EU58; A0A0F7UR23; A0A0F7UX68; S8F957; A0A7J6KDD8; A0A086JB35; A0A3R7YIL7; A0A2G8XM30; A0A139XIH1; A0A2T6IDA8; A0A151GYW9; A0A086PJ28; S7UHE2 and A0A086J6J6 (Fig. S1B). Finally, one peptide (VNAEALDLDR) was homology to a third group of 8 OTU-like cysteine protease domain-containing proteins (group 3) of MW ~ 22 kDa and accession numbers of: S7V2A4; A0A7J6K361; A0A3R8AIE5; A0A2T6J5G6; A0A086QEF7; A0A086LFR6; A0A086L2L9 and A0A086KVA5 (Fig. S1C). Collectively, these data suggested that protease from S. fusiformis cyst might be related to the OTU-like cysteine protease domain-containing proteins.
Figure 6 depicts the influence of various pH and temperature values on the proteolytic activity of the purified 0.2 M NaCl-pooled fraction. The purified protease showed high enzyme activities in pH values ranging from 6.0 to 7.0 with an optimum pH of 6.0 (Fig. 6A). The purified protease displayed its optimal activity at 50 °C (Fig. 6B). And for confirmation, the enzyme activity has been measured over different time periods (15–90 min) at 50 °C using the caseinolytic standard assay. The results showed that the purified enzyme retained 100% of its activity when incubated with the substrate at 50 °C for 75 min then a very slight decrease (5%) was noticed at 90 min (Fig.6C). Therefore, this temperature (50 °C) was selected throughout the experiments as the standard temperature. For thermal stability, the purified enzyme maintained 100% activity when it was incubated alone for 30 min up to 40 °C; however, at 50 and 60 °C, a minor drop-in activity was observed (Fig. 6D). In addition, 47% of the enzyme's activity was still present at 70 °C. It could be concluded that the purified enzyme is a thermostable enzyme with optimal activity at 50 °C and pH 6.0.
(A) Optimum-pH of the purified protease, (B) Optimum-temperature of the protease activity, (C) Relative activity % of the purified protease over different time periods (15–90 min) at 50 °C using the caseinolytic standard assay, (D) Thermal stability of the purified enzyme. The enzyme was incubated for 30 min at various temperatures (25–70 °C) before adding the substrate. After that, it was cooled in an ice bath. The residual activity was assessed using the caseinolytic standard assay. The values represent mean ± SD (n = 3).
Under ideal conditions (pH 6.0 and 50 °C), the kinetic properties of the purified-protease were examined using various substrate concentrations. Figure 7 shows the graphically determined Km, Vmax and Kcat values which were 0.018 mg/ml, 52.54 U/ml and 3.6 × 106 S−1, respectively. A low Km value indicates the high affinity of the purified-enzyme toward the substrate.
Michaelis–Menten plot of the purified protease using azocasein as a substrate at a concentration range of 0.025–0.20 mg/ml and reaction rate (velocity) has been measured under standard assay conditions.
The activity of the purified enzyme was assessed in the presence of several protease inhibitors using a specific cysteine protease substrate (BAPNA-HCl) as shown in Table 4. At a concentration of 2 and 5 mM, the cysteine protease inhibitors, p-choromeruic benzoic acid, iodoacetic acid, and N-ethylmaleimide, totally suppressed the enzyme activity. While the metalloprotease-inhibitors (EDTA and o-phenanthroline) and serine protease-inhibitors (Soybean trypsin inhibitor and PMSF) have no inhibitory effect on the enzyme activity. From this finding, the purified-protease enzyme belongs to the cysteine protease group. Herein, the influence of various metal ions on enzyme activity was also investigated. At 2 and 5 mM, Zn2+, Ni2+, Hg2+, and Cu2+ act as potent inhibitors of the enzyme's activity, whereas the Ca2+ and Mg2+ have no impact on the enzyme's activity (Table 5).
The proteolytic activity of the purified enzyme was assessed in the presence of a variety of soluble and insoluble protein substrates (Table 6). A significant selectivity was observed for the insoluble substrates, including fibrin, native collagen I, and hemoglobin, with relative activity percentages of 100, 81, and 53%, respectively. For the soluble substrates, gelatin (denatured collagen) was preferable over casein and albumin with relative activity percentages of 43, 22, and 15%, respectively. This observation suggests that the purified protease has a high affinity towards blood and capillary wall components, resulting in disruption of the capillary wall and extravasations.
Numerous investigations revealed that the Sarcocystis spp. infection rate in sheep, cattle, and buffaloes is high worldwide6,12,13,14,28,29,30. Here, macroscopic and histological examinations were performed on 19 samples isolated from infected buffaloes collected at the El-Basateen slaughterhouse, Cairo province. The observation revealed that all animals were infected with S. fusiforms. Molecular analyses on the genetic characterization of diverse Sarcocystis spp. are usually carried out on target genes such as ITS, COX1, and 18S rRNA31,32,33. For accurate species identification, we used molecular techniques to confirm Sarcocystis species recognition in 15 samples. It is clear from the 18SrRNA, ITS1, and Cox1 phylogeny trees that the Sarcocystis found in Cairo province is identical to the sequences of S. fusiformis. Those sequences were previously reported in India, Egypt, and China. The characterization using the 18S gene revealed a marked similarity between the water buffalo species S. fusiformis and others in the Egyptian buffalo species34, the African buffalo species S. cafferi, and the cattle species S. hirsute33. S. fusiformis species was found in buffaloes in various Provinces throughout Egypt by several authors9,13,34,35,36. However, this species has been documented in water buffaloes over the world, including India37,38, China39, Iraqi35, Vietnam40 and Iran41. Current results and other previous findings suggest that S. fusiformis may be considered one of the most prevalent species of sarcocystis found in Egyptian buffaloes.
During the parasite's invasion of the host, proteases from protozoa perform a variety of functions. Dissolution of the extracellular matrix of the host is one of these, and it helps the parasite get to the surface of the host cell. In addition, protozoan proteases actively participate in the breakdown of host immune molecules such as immunoglobulins, making it possible for the pathogen to escape immune responses from the host. The protozoan protease also performs cytolysis and phagocytosis on target cells42. Cysteine proteases sometimes referred to as papain-like or thiol proteases possess a His/Cys catalytic dyad that interacts with one another. The cysteine (sulfhydryl group) initiates an attack on the peptide bond's carbonyl carbon during proteolysis by acting as a nucleophile. It has been discovered that parasitic protozoan cysteine proteases are involved in all of the major stages of disease development43. The merozoites of S. muris contained several basic and four acidic proteases. One of those basic proteases was further characterized as a thiol protease. The merozoite dense granules, the organelle involved in the parasite invasion of the host cell, were rich in thiol protease44. Therefore, the present study focused on purifying and characterizing proteases from S. fusiforms cysts. One-hundred kDa of protease was purified from S. fusiform cysts to homogeneity by two steps of purification with a yield of 42.6%.
This is the first study to our knowledge to describe the biochemical properties of the protease from S. fusiformis. Five identified peptide fragments of the purified protease-enzyme structure are highly similar to the N- and C-terminal ends of Toxoplasma cysteine protease and ovarian tumor unit domain-containing cysteine protease. This identification indicates that the purified protease belongs to the cysteine proteases. Additionally, the cysteine protease inhibitors completely blocked the purified enzyme activity, which was not impacted by serine and metalloprotease inhibitors. So, it is categorized as a cysteine protease. Similar results were reported for cysteine proteases from Fasiola gigantica45 and Triticum aestivum46. Further, the heavy metal ions Zn2+, Ni2+, Hg2+, and Cu2+ are effective enzyme inhibitors, whereas Ca2+ and Mg2+ have no impact on the enzyme's activity. Most of the tested heavy metal ions inhibited the cysteine proteases that were purified from Fasiola gigantica45 and Triticum aestivum46. Hg2+ poisoning is commonly attributed to the strong interactions that exist between Hg2+ and cysteine thiolate anions in cysteine proteases47. Moreover, the purified cysteine protease retained most activity in the pH range (6–7) and temperature range (40–50 °C). Several studies reported that S. neurona merozoites had serine protease activity with an optimal pH of 8 to 10 and a relative molecular weight of 65–70 kDa. And S. neurona merozoites were significantly prevented from entering cells by the serine inhibitors. Toxoplasma gondii had five genes encoding cathepsins (cysteine protease). Almost all T. gondii cathepsin proteases function best in low pH ranges (5.5–6.5), and they are differentially expressed in all parasitic stages of T. gondii (tachyzoites, bradyzoites, and sporozoites)48,49,50. T. gondii tachyzoite invasion was significantly reduced by PRT2253, a specific cysteine protease inhibitor of cathepsin-B51. Therefore, this similar characterization showed that T. gondii cysteine proteases were similar to S. fusiform cysteine proteases.
It has been reported that the optimum temperature of proteases from Plasmodium knowlesi and Fasciola gigantica was detected at 50 °C52,53. Additionally, it was shown that some Plasmodium parasite recombinant enzymes function best at temperatures between 50 and 60 °C54. The results of the current work observed that S. fusiform protease showed the same maximum catalytic activity at 50 °C. Interestingly, S. fusiform protease exhibited thermostability at a temperature of 40 °C and a slight decrease in activity at 50 °C for 30 min of incubation. It shares the character of thermostability with 80 kDa metalloprotease from T. gondii, possessing stability at 37 °C and a slight decrease at 56 °C for 30 min incubation55. Mathews et al.56 emphasized that the parasite encountered a series of thermal stresses during its entry into the host, like recurrent episodes of fever manifested in the host (reaching 41 °C or greater). The thermal stability of S. fusiform protease might be correlated with the parasite’s need to survive under extreme temperature changes in the environment. Further, the current study also indicated that the S. fusiform protease had a Km value of 0.018 mg azocasein/mL and Vmax value of 52.54 U/ml. In contrast, higher Km values were reported for proteases from F. gigantica (5 mg azocasein/ml)52 and microbe (3.8 azocasein/ml)57.
Current research demonstrated that S. fusiform protease effectively hydrolyzed several mammalian proteins, including fibrin and collagen I, albumin, hemoglobin, and gelatin. However, the enzyme showed strong activity against fibrin and collagen I. Sarcocystis forms observable cysts containing numerous undeveloped individuals. The cysts offer defense against the immune system, nourishment, and proper conditions of temperature, and hydration7,58,59. Several cathepsins from trematodes have anticoagulant potential due to their ability to effectively cleave fibrin and fibrinogen, promoting blood feeding60. They are also involved in the degradation of collagen, which is an important constituent of the host tissue61. Plasmodium parasites consume and digest host hemoglobin within the host cell niche to obtain the amino acids necessary for protein synthesis62. Falcipains, the major cysteine proteases of P. falciparum, were expressed in P. falciparum stages, and they efficiently hydrolyze hemoglobin at food vacuolar pH (approximately pH 5.5). Falcipains were impeded by cysteine protease inhibitors, which inhibit hemoglobin breakdown and parasite maturation63. Based on the displayed data, S. fusiform cysteine protease may be involved in invasion and intracellular growth.
The current work reports the identification of Sarcocystis fusiformis from infected Egyptian water buffaloes based on histological observation and molecular analysis. The biochemical characterization of S. fusiform protease and the data on protein sequence suggested that protease might be related to the OTU-like cysteine protease. The results demonstrated unequivocally that the cysteine protease released by S. fusiform plays a crucial role in the behavior of parasites during tissue invasion, which is crucial for parasite survival. As a result, this enzyme may severely affect parasite viability if it is inactivated, making it a suitable target for chemotherapeutic drugs or vaccinations.
The datasets generated during and/or analyzed during the current study are available from the corresponding author on reasonable request.
Fayer, R., Esposito, D. H. & Dubey, J. P. Human infections with Sarcocystis species. Clin. Microbiol. Rev. 28(2), 295–311 (2015).
PubMed PubMed Central Google Scholar
Verma, S. K., Lindsay, D. S., Grigg, M. E. & Dubey, J. P. Isolation, culture and cryopreservation of Sarcocystis species. Curr. Protoc. Microbiol. 45, 20d.21.21-20d.21.27 (2017).
Daryani, A. et al. Survey of Sarcocystis infection in slaughtered sheep and buffaloes in Ardabil, Iran. J. Anim. Vet. Adv. 5, 60–62 (2006).
Ayazian Mavi, S. et al. Sarcocystis infection in beef and industrial raw beef burgers from butcheries and retail stores: A molecular microscopic study. Heliyon 6(6), e04171 (2020).
PubMed PubMed Central Google Scholar
Fayer, R. Sarcocystis spp. in human infections. Clin. Microbiol. Rev. 17(4), 894–902 (2004).
PubMed PubMed Central Google Scholar
Bahari, P., Salehi, M., Seyyedabadi, M. & Mohammadi, A. Molecular identification of macroscopic and microscopic cysts of Sarcocystis in sheep in North Khorasan Province, Iran. Int. J. Mol. Cell. Med. 3(1), 51–56 (2014).
CAS PubMed PubMed Central Google Scholar
Dubey, J. P., Speer, C. & Fayer, R. Sarcocystosis of Animals and Man (CRC Press, 1989).
Latif, B. M., Al-Delemi, J. K., Mohammed, B. S., Al-Bayati, S. M. & Al-Amiry, A. M. Prevalence of Sarcocystis spp. in meat-producing animals in Iraq. Vet. Parasitol. 84(1–2), 85–90 (1999).
El-Bahy, N., El-Bagory, A.-E.-R., AbouLaila, M., Elkhatam, A. & Mady, H. M. Prevalence of Sarcocystis fusiformis and hydatid cyst among different ruminants at Menofia Governorate, Egypt. J. Curr. Vet. Res. 1(1), 1–10 (2019).
Hilali, M., El-Seify, M., Zayed, A., El-Morsey, A. & Dubey, J. P. Sarcocystis dubeyi (Huong and Uggla, 1999) infection in water buffaloes (Bubalus bubalis) from Egypt. J. Parasitol. 97(3), 527–528 (2011).
Abu-Elwafa, S., Al-Araby, M. & Abbas, I. Comparative ultrastructure of two types of Sarcocystis spp. of water buffaloes (Bubalus bubalis) from Egypt. In Proceedings of the 5th Scientific Conference of Animal Wealth Research in the Middle East and North Africa, Faculty of Agriculture, Cairo University, Giza, Egypt, 1–3 October 2012. Massive Conferences and Trade Fairs 48–59 (2012).
Ashmawy, K. I., Abu-Akkada, S. S. & Ghashir, M. B. Prevalence and molecular characterization of Sarcocystis species in water buffaloes (Bubalus bubalus) in Egypt. Trop. Anim. Health Prod. 46(8), 1351–1356 (2014).
Ras, R. A. Prevalence and molecular identification of Sarcocystis spp. infecting water buffaloes (Bubalus bubalus) in Sharkia Province, Egypt. Egypt. Vet. Med. Soc. Parasitol. J. (EVMSPJ) 17(1), 1–19 (2021).
Gerab, R. A., Edris, A.-B.M., Lamada, H. M. & Elrais, A. Prevalence and distribution of Sarcocystis in buffaloes and sheep in Egypt. J. Adv. Vet. Res. 12(3), 302–307 (2022).
Wes Leid, R. & Suquet, C. M. A superoxide dismutase of metacestodes of Taenia taeniaeformis. Mol. Biochem. Parasitol. 18(3), 301–311 (1986).
Seetharam, R. & Sharma, S. K. Purification and Analysis of Recombinant Proteins Vol. 12 (CRC Press, 1991).
Ekici, O. D., Paetzel, M. & Dalbey, R. E. Unconventional serine proteases: Variations on the catalytic Ser/His/Asp triad configuration. Protein Sci. 17(12), 2023–2037 (2008).
CAS PubMed PubMed Central Google Scholar
Perkins, P. S., Haley, D. & Rosenblatt, R. Proteolytic enzymes in the blood-feeding parasitic copepod, Phrixocephalus cincinnatus. J. Parasitol. 83(1), 6–12 (1997).
Shaw, M. K., Roos, D. S. & Tilney, L. G. Cysteine and serine protease inhibitors block intracellular development and disrupt the secretory pathway of Toxoplasma gondii. Microbes Infect. 4(2), 119–132 (2002).
McKerrow, J. H., Rosenthal, P. J., Swenerton, R. & Doyle, P. Development of protease inhibitors for protozoan infections. Curr. Opin. Infect. Dis. 21(6), 668–672 (2008).
CAS PubMed PubMed Central Google Scholar
Chaimon, S. et al. Molecular characterization and functional analysis of the Schistosoma mekongi Ca2+-dependent cysteine protease (calpain). Parasit. Vectors 12(1), 383 (2019).
PubMed PubMed Central Google Scholar
Yan, W. et al. Morphological and molecular characterization of Sarcocystis miescheriana from pigs in the central region of China. Parasitol. Res. 112(3), 975–980 (2013).
Lemos, F. J. A., Campos, F. A. P., Silva, C. P. & Xavier-Filho, J. Proteinases and amylases of larval midgut of Zabrotes subfasciatus reared on cowpea (Vigna unguiculata) seeds. Entomol. Exp. Appl. 56(3), 219–227 (1990).
Lin, X., Shih, J. & Swaisgood, H. E. Hydrolysis of feather keratin by immobilized keratinase. Appl. Environ. Microbiol. 62(11), 4273–4275 (1996).
CAS ADS PubMed PubMed Central Google Scholar
Arnon, R. Papain. Methods Enzymol. 19, 226–244 (1970).
Bradford, M. M. A rapid and sensitive method for the quantitation of microgram quantities of protein utilizing the principle of protein-dye binding. Anal. Biochem. 72, 248–254 (1976).
Laemmli, U. K. Cleavage of structural proteins during the assembly of the head of bacteriophage T4. Nature 227(5259), 680–685 (1970).
CAS ADS PubMed Google Scholar
Portella, L. P. et al. Molecular detection and characterization of Sarcocystis infection in naturally infected buffaloes, Brazil. J. Food Prot. 84(3), 429–433 (2021).
Dessì, G. et al. A survey on Apicomplexa protozoa in sheep slaughtered for human consumption. Parasitol. Res. 121(5), 1437–1445 (2022).
PubMed PubMed Central Google Scholar
Phythian, C. J. et al. Abattoir surveillance of Sarcocystis spp., Cysticercosis ovis and Echinococcus granulosus in Tasmanian slaughter sheep, 2007–2013. Aust. Vet. J. 96(3), 62–68 (2018).
Jehle, C. et al. Diagnosis of Sarcocystis spp. in cattle (Bos taurus) and water buffalo (Bubalus bubalis) in Northern Vietnam. Vet. Parasitol. 166(3), 314–320 (2009).
Gjerde, B., Hilali, M. & Abbas, I. E. Molecular differentiation of Sarcocystis buffalonis and Sarcocystis levinei in water buffaloes (Bubalus bubalis) from Sarcocystis hirsuta and Sarcocystis cruzi in cattle (Bos taurus). Parasitol. Res. 115(6), 2459–2471 (2016).
Sudan, V., Kumar, R., Shanker, D. & Singh, A. Sequence phylogenetic analysis and associative genetic diversity of Sarcocystis hirsuta based on 18S rRNA gene. Beni-Suef Univ. J. Basic Appl. Sci. 10(1), 22 (2021).
El-Seify, M. et al. Molecular characterization of Sarcocytis fusiformis and Sarcocystis buffalonis infecting water buffaloes (Bubalus bubalis) from Egypt. Am. J. Anim. Vet. Sci. 9(2), 95–104 (2014).
Dakhil, H., Abdallah, H. & Abdullah, F. Molecular identification of Sarcocystis fusiformis and S. moulei infecting water buffaloes (Bubalus bubalis) in Southern Iraq. World J. Pharm. Res. 6, 215–229 (2017).
Gjerde, B., Hilali, M. & Mawgood, S. A. Molecular characterisation of three regions of the nuclear ribosomal DNA unit and the mitochondrial cox1 gene of Sarcocystis fusiformis from water buffaloes (Bubalus bubalis) in Egypt. Parasitol. Res. 114(9), 3401–3413 (2015).
JyothiSree, C., Venu, R., Samatha, V., Malakondaiah, P. & Rayulu, V. C. Prevalence and microscopic studies of Sarcocystis infection in naturally infected water buffaloes (Bubalus bubalis) of Andhra Pradesh. J. Parasit. Dis. 41(2), 476–482 (2017).
Sudan, V., Shanker, D., Paliwal, S., Kumar, R. & Singh, A. Phylogenetics of Sarcocystis fusiformis isolates based on 18S rRNA and cox 1 genes. Microb. Pathog. 159, 105144 (2021).
Yang, Z. Q. et al. Analysis of the 18S rRNA genes of Sarcocystis species suggests that the morphologically similar organisms from cattle and water buffalo should be considered the same species. Mol. Biochem. Parasitol. 115(2), 283–288 (2001).
Jehle, C. et al. Diagnosis of Sarcocystis spp. in cattle (Bos taurus) and water buffalo (Bubalus bubalis) in Northern Vietnam. Vet. Parasitol. 166(3–4), 314–320 (2009).
Oryan, A., Ahmadi, N. & Mousavi, S. M. Prevalence, biology, and distribution pattern of Sarcocystis infection in water buffalo (Bubalus bubalis) in Iran. Trop. Anim. Health Prod. 42(7), 1513–1518 (2010).
Ghosh, A. & Raha, S. Proteases from protozoa and their role in infection. In Proteases in Physiology and Pathology (eds Chakraborti, S. & Dhalla, N. S.) 143–158 (Springer Singapore, 2017).
Verma, S., Dixit, R. & Pandey, K. C. Cysteine proteases: Modes of activation and future prospects as pharmacological targets. Front. Pharmacol. 7, 107. https://doi.org/10.3389/fphar.2016.00107 (2016).
Article CAS PubMed PubMed Central Google Scholar
Strobel, J. G., Delplace, P., Dubremetz, J.-F. & Entzeroth, R. Sarcocystis muris (Apicomplexa): A thiol protease from the dense granules. Exp. Parasitol. 74(1), 100–105 (1992).
Mohamed, S. A., Fahmy, A. S., Mohamed, T. M. & Hamdy, S. M. Proteases in egg, miracidium, and adult of Fasciola gigantica. Characterization of serine and cysteine proteases from adult. Comp. Biochem. Physiol. Part B 142, 192–200 (2005).
Fahmy, A. S., Ali, A. A. & Mohamed, S. A. Characterization of a cysteine protease from wheat Triticum aestivum (cv. Giza 164). Bioresour. Technol. 91, 297–304 (2004).
Stratton, A., Ericksen, M., Harris, T. V. & Symmonds, N. Mercury (II) binds to both of chymotrypsin’s histidines, causing inhibition followed by irreversible denaturation/aggregation. Protein Sci. 26, 292–305 (2017).
CAS PubMed PubMed Central Google Scholar
Que, X. et al. Cathepsin Cs are key for the intracellular survival of the protozoan parasite, Toxoplasma gondii. J. Biol. Chem. 282(7), 4994–5003 (2007).
Huang, R. et al. The cathepsin L of Toxoplasma gondii (TgCPL) and its endogenous macromolecular inhibitor, toxostatin. Mol. Biochem. Parasitol. 164(1), 86–94 (2009).
Parussini, F., Coppens, I., Shah, P. P., Diamond, S. L. & Carruthers, V. B. Cathepsin L occupies a vacuolar compartment and is a protein maturase within the endo/exocytic system of Toxoplasma gondii. Mol. Microbiol. 76(6), 1340–1357 (2010).
CAS PubMed PubMed Central Google Scholar
Que, X. et al. The cathepsin B of Toxoplasma gondii, toxopain-1, is critical for parasite invasion and rhoptry protein processing. J. Biol. Chem. 277(28), 25791–25797 (2002).
Mohamed, S. A., Fahmy, A. S., Mohamed, T. M. & Hamdy, S. M. Proteases in egg, miracidium and adult of Fasciola gigantica. Characterization of serine and cysteine proteases from adult. Comp. Biochem. Physiol. Part B Biochem. Mol. Biol. 142(2), 192–200 (2005).
Budiman, C., Razak, R. A., Unggit, A. R. A. & Razali, R. Catalytic Properties of caseinolytic protease subunit of Plasmodium knowlesi and its inhibition by a member of δ-lactone, hyptolide. Molecules 27(12), 3787 (2022).
CAS PubMed PubMed Central Google Scholar
Crowther, G. J. et al. Use of thermal melt curves to assess the quality of enzyme preparations. Anal. Biochem. 399(2), 268–275 (2010).
Song, K. J. & Nam, H. W. Protease activity of 80 kDa protein secreted from the apicomplexan parasite Toxoplasma gondii. Korean J. Parasitol. 41(3), 165–169 (2003).
PubMed PubMed Central Google Scholar
Mathews, E. S., Jezewski, A. J. & Odom John, A. R. Protein prenylation and Hsp40 in thermotolerance of Plasmodium falciparum malaria parasites. MBio 12(3), e00760-00721 (2021).
Biver, S., Portetelle, D. & Vandenbol, M. Characterization of a new oxidant-stable serine protease isolated by functional metagenomics. Springerplus 2(1), 410 (2013).
PubMed PubMed Central Google Scholar
Jäkel, T. et al. Reduction of transmission stages concomitant with increased host immune responses to hypervirulent Sarcocystis singaporensis, and natural selection for intermediate virulence. Int. J. Parasitol. 31, 1639–1647 (2002).
Kojouri, G., Aghajani, E., Jahanabadi, S. & Kojouri, A. Mineral status of myocardial sarcocystosis. Iran. J. Parasitol. 6(2), 17–22 (2011).
CAS PubMed PubMed Central Google Scholar
Mebius, M. M. et al. Fibrinogen and fibrin are novel substrates for Fasciola hepatica cathepsin L peptidases. Mol. Biochem. Parasitol. 221, 10–13 (2018).
Robinson, M. W. et al. Collagenolytic activities of the major secreted cathepsin L peptidases involved in the virulence of the helminth pathogen, Fasciola hepatica. PLoS Negl. Trop. Dis. 5(4), e1012 (2011).
CAS PubMed PubMed Central Google Scholar
Counihan, N. A., Modak, J. K. & de Koning-Ward, T. F. How malaria parasites acquire nutrients from their host. Front. Cell Dev. Biol. 9, 649184 (2021).
PubMed PubMed Central Google Scholar
Prasad, R., Atul, Soni, A., Puri, S. K. & Sijwali, P. S. Expression, Characterization, and cellular localization of knowpains, papain-like cysteine proteases of the plasmodium knowlesi malaria parasite. PLoS One 7(12), e51619 (2012).
CAS ADS PubMed PubMed Central Google Scholar
This paper is based on work supported by Science, Technology and Innovation Funding Authority (STDF) under grant (BASIC AND APPLIED SCIENCE RESEARCHS CALL-7) Code No. 38201.
Open access funding provided by The Science, Technology & Innovation Funding Authority (STDF) in cooperation with The Egyptian Knowledge Bank (EKB).
Molecular Biology Department, National Research Centre, Dokki, Cairo, Egypt
Amal Z. Barakat, Azza M. Abdel-Aty, Hala A. Salah, Usama M. Hegazy, Rasha AM Azouz, Roqaya I. Bassuiny & Saleh A. Mohamed
Department of Microbial Biotechnology, National Research Centre, Dokki, Cairo, Egypt
Zoonotic Disease Department, National Research Centre, Dokki, Cairo, Egypt
You can also search for this author in PubMed Google Scholar
You can also search for this author in PubMed Google Scholar
You can also search for this author in PubMed Google Scholar
You can also search for this author in PubMed Google Scholar
You can also search for this author in PubMed Google Scholar
You can also search for this author in PubMed Google Scholar
You can also search for this author in PubMed Google Scholar
You can also search for this author in PubMed Google Scholar
You can also search for this author in PubMed Google Scholar
A.Z.B., S.A.M. and A.M.A. designed the research; M.K.I., H.A.S., U.M.H., R.A.M.A., R.I.B. and R.M.S. conducted the research; A.Z.B., S.A.M., A.M.A. and U.M.H. analyzed the data; A.Z.B., S.A.M. and A.M.A. wrote the paper. All authors have read and approved the final manuscript.
Correspondence to Amal Z. Barakat or Saleh A. Mohamed.
The authors declare no competing interests.
Springer Nature remains neutral with regard to jurisdictional claims in published maps and institutional affiliations.
Open Access This article is licensed under a Creative Commons Attribution 4.0 International License, which permits use, sharing, adaptation, distribution and reproduction in any medium or format, as long as you give appropriate credit to the original author(s) and the source, provide a link to the Creative Commons licence, and indicate if changes were made. The images or other third party material in this article are included in the article's Creative Commons licence, unless indicated otherwise in a credit line to the material. If material is not included in the article's Creative Commons licence and your intended use is not permitted by statutory regulation or exceeds the permitted use, you will need to obtain permission directly from the copyright holder. To view a copy of this licence, visit http://creativecommons.org/licenses/by/4.0/.
Barakat, A.Z., Abdel-Aty, A.M., Ibrahim, M.K. et al. Purification and characterization of cysteine protease of Sarcocystis fusiformis from infected Egyptian water buffaloes. Sci Rep 13, 16123 (2023). https://doi.org/10.1038/s41598-023-43147-1
DOI: https://doi.org/10.1038/s41598-023-43147-1
Anyone you share the following link with will be able to read this content:
Sorry, a shareable link is not currently available for this article.
Provided by the Springer Nature SharedIt content-sharing initiative
By submitting a comment you agree to abide by our Terms and Community Guidelines. If you find something abusive or that does not comply with our terms or guidelines please flag it as inappropriate.
Scientific Reports (Sci Rep) ISSN 2045-2322 (online)
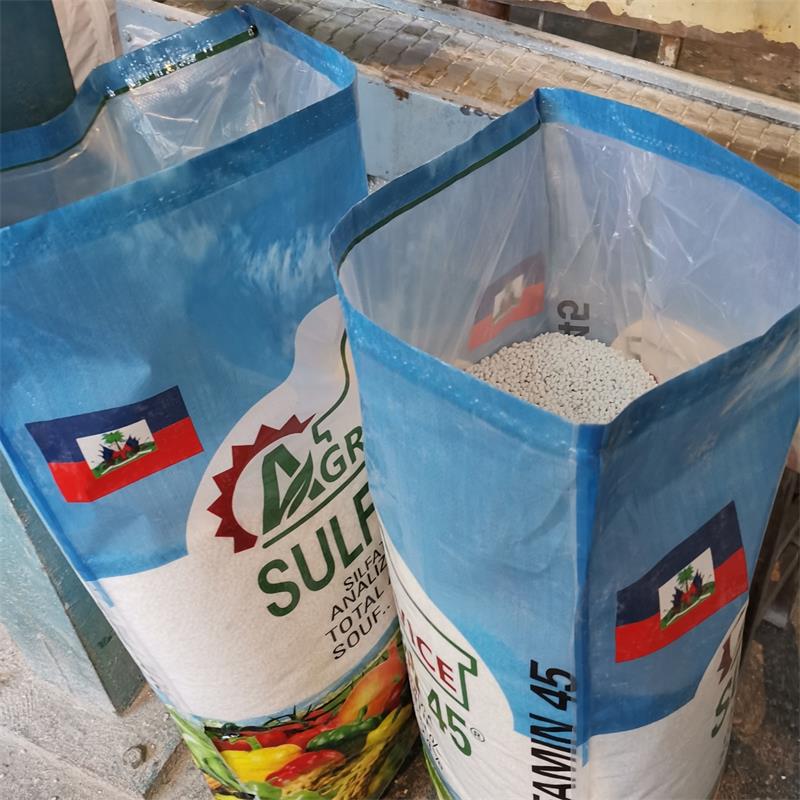
Liquid Ammonium Sulfate Sign up for the Nature Briefing newsletter — what matters in science, free to your inbox daily.