Thank you for visiting nature.com. You are using a browser version with limited support for CSS. To obtain the best experience, we recommend you use a more up to date browser (or turn off compatibility mode in Internet Explorer). In the meantime, to ensure continued support, we are displaying the site without styles and JavaScript.
Scientific Reports volume 14, Article number: 516 (2024 ) Cite this article Botanical Active Ingredient
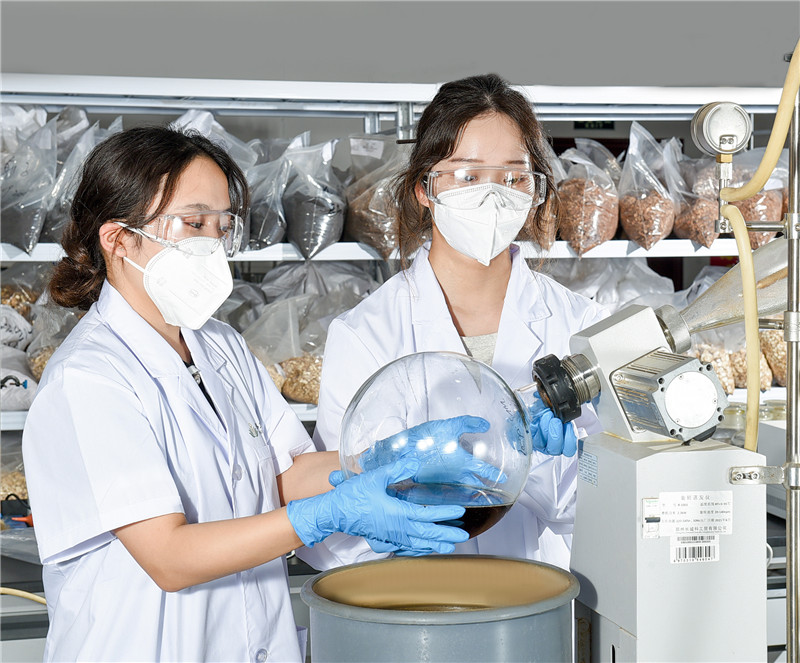
To explore the anti-tumor effects of Radix Astragali on hypopharyngeal carcinoma and its mechanism. We have bioinformatically analyzed the potential targets of Radix Astragali and predicted the molecular mechanism of Radix Astragali treating of hypopharyngeal carcinoma. The binding process of the hub targets that could prolong the survival time of hypopharyngeal cancer patients with Radix Astragali was simulated by molecular docking. The results showed that 17 out of 36 hub targets could effectively improve the 5-year survival rate of hypopharyngeal cancer patients. Radix Astragali acts on hypopharyngeal carcinoma by regulating a signaling network formed by hub targets connecting multiple signaling pathways and is expected to become a drug for treating and prolonging hypopharyngeal carcinoma patients’ survival time.
Cancers of the hypopharynx are relatively rare, accounting for about 3% of all head and neck cancers1. Unfortunately, hypopharyngeal cancers have the worst prognosis of all head and neck cancers, with 5-year overall survival rates as low as 30–35%2,3. The cause of hypopharyngeal cancer is unclear and may be related to alcoholism, smoking, and viral infections (HPV, EBV)4. Due to its unique anatomical location, hypopharyngeal cancer has an insidious onset in the early stage. It often progresses to the middle or late stage when clinical symptoms appear, and its diagnosis relies on laryngoscopy, esophageal angiography, enhanced CT and MRI, etc. Its treatment mainly consists of surgery combined with radiotherapy and chemotherapy. However, due to the high degree of malignant and invasive hypopharyngeal cancer, the ease of lymphatic metastasis in the early stage, the early symptomatology is atypical, and the poor sensitivity of radiotherapy and chemotherapy, etc., which leads to unsatisfactory prognostic results and the 5-year survival rate of hypopharyngeal cancer is less than 30%5. As the pace of laryngeal preservation in advanced patients undergoing surgical treatment is low, and various complications such as pharyngeal fistula and ergotism will reduce the quality of survival and increase the burden of life of the patients, it is of great significance to search for new therapeutic options for hypopharyngeal cancer to improve the survival rate of patients with hypopharyngeal carcinoma.
Radix Astragali is one of the traditional Chinese medicines6. Traditional Chinese medicines are now widely used in clinical treatment7, both to act on a variety of targets8,9 to inhibit cancer and to alleviate the adverse effects of anticancer drugs10. Radix Astragali is widely used for its unique medicinal value and health benefits and is considered a traditional medicine and food11. In the toxicity study of Radix Astragali membranaceus extract, acute and subchronic oral toxicity tests were conducted in rats. In the critical toxicity study, a single dose of up to 5000 mg/kg was administered. In a 13-week subchronic toxicity study based on clinical signs, body weights, and necropsy findings, there were no deaths or toxic reactions12, which illustrates the safety of the Radix Astragali application. Whereas the interaction of Radix Astragali with other drugs may improve efficacy, astragaloside combined with apatinib mesylate significantly inhibited the growth of hepatocellular carcinoma. It promoted apoptosis13 and the combination with doxorubicin reduced myocardial toxicity14. It is extensively documented that the active ingredients of Radix Astragali affect various tumor biological behaviors, such as proliferation, apoptosis, migration, and infiltration15,16,17. As one of the bioactive substances of Radix Astragali, Radix Astragali polysaccharide (APS) significantly blocked the wnt/β-linker protein signaling pathway in a dose-dependent manner and thus inhibited the proliferation of breast cancer cells18. APS also inhibited epithelial-to-mesenchymal transition (EMT) and suppressed the expression of RAS/ERK and MAPK/NFkB pathway-related proteins in MSC-induced lung cancer cells19. APS is just one of the studies on biologically active substances related to anticancer in Radix Astragali. Astragaloside (AST) activates the pro-apoptotic pathway in colorectal cancer (CRC) cell lines, as evidenced by a decrease in the expression of the anti-apoptotic proteins Bcl-2, PARP and cysteinyl asparaginase 3 and an increase the expression of the pro-apoptotic proteins Bax, Bak, and Bad20. In the study of head and neck squamous cell carcinoma, it was found that Radix Astragali induced apoptosis in nasopharyngeal carcinoma cells, as evidenced by the elevated levels of cysteinyl asparaginase and Bax protein and the decreased level of Bcl-2 protein21, and APS significantly promoted the antiproliferative and apoptotic effects of cisplatin on nasopharyngeal carcinoma cells22. However, the literature has not reported its impact on hypopharyngeal carcinoma.
Based on systems biology, computer science, and bioinformatics, network pharmacology can efficiently and cost-effectively explore the relationship between drugs and diseases through high-throughput technologies, which enables the transition from the single-drug-single-target model of traditional pharmacology to the multi-drug-multi-target model23,24,25,26. Therefore, in this study, the effects of Radix Astragali on hypopharyngeal carcinoma and its specific mechanisms were systematically investigated using network pharmacology and molecular docking techniques.
The active ingredients of Radix Astragali were searched in the TCMSP database using the keyword “Radix Astragali”. The search criteria of OB > 30% and DL > 0.08 were used for the preliminary screening of the active ingredients of Radix Astragali. The obtained active ingredients were used as the effective active ingredients of Radix Astragali for subsequent data processing (OB and DL were selected according to the TCMSP website drug screening criteria recommendations and adjusted according to how many Radix Astragali targets were retrieved). TCMSP is a platform on the systemic pharmacology of herbal medicines, where we can get the relationship between drug, target and disease. The database provides information on identifying active ingredients, drug target networks, associated drug-target-disease networks, and pharmacokinetic properties such as oral bioavailability (OB)27 and drug-likeness (DL)28 for natural compounds. OB represents the percentage of an orally administered dose of an unchanged drug that reaches the systemic circulation, revealing the ADME process’s convergence. High oral bioavailability is often a key indicator to determine the drug-like properties of bioactive molecules as therapeutic agents. DL is a qualitative concept used in drug design to estimate how “drug-like” a prospective compound is, which helps optimize pharmacokinetic and pharmaceutical properties, such as solubility and chemical stability. The ‘drug-like’ level of the compounds is 0.18, which is used as a selection criterion for the ‘drug-like’ compounds in traditional Chinese herbs.
Based on the active ingredients of Radix Astragali, Radix Astragali-related targets were screened in the TCMSP database, and the targets were searched in the ETCM database and Symmap database, respectively with “Radix Astragali” as the keyword and the targets obtained from the above three databases were integrated and de-duplicated. The targets obtained were regarded as Radix Astragali-related targets. ETCM is a comprehensive resource database of Chinese herbal medicines that went online in 2018 by the Chinese Academy of Traditional Chinese Medicine, bringing together information on a wide range of herbal medicines, herbal compounding, herbal chemical composition, drug targets, and related diseases. SymMap (Symptom Mapping) is a Chinese medicine evidence association database. The database herbs and the corresponding traditional Chinese medicine symptoms and correspond classic Chinese medicine symptoms to Western medicine symptoms, and includes diseases, herbal ingredients, drug targets associated with these symptoms, as well as the correlations between these six data types.
We searched the Genecard database for hypopharyngeal carcinoma-related targets using the keyword “Hypopharyngeal Carcinoma”. The targets obtained were hypopharyngeal carcinoma-related targets. Genecards is a comprehensive, searchable database of genes where we can access information on almost all known human genes. Genecards automatically integrates resources from approximately 150 gene-centric databases, including genomics, transcriptomics, proteomics, genetics, clinical and functional information, and more.
Venn diagram of Radix Astragali-related targets and hypopharyngeal carcinoma-related targets were plotted using R software (R 4.2.0), and the intersecting targets were used as potential targets of Radix Astragali for hypopharyngeal carcinoma. Then, we imported the potential targets of Radix Astragali for hypopharyngeal carcinoma into the String database, set the species as “Homo sapiens”, and set the confidence level as “0.9” to draw the PPI network.
We imported the PPI network into Cytoscape software (3.8.0) to further analyze the coefficients of the obtained marks, such as degree, Betweenness Centrality, and Closeness Centrality, and then, using degree ≥ degree median, Betweenness Centrality ≥ Closeness Centrality median and Closeness Centrality median as the screening criteria to screen the hub targets. The obtained targets were the hub targets.
We imported the hub targets into the Kaplan–Meier Plotter online platform to plot KM curves. Since the platform does not have a separate database for Hypopharyngeal Carcinoma, we based the KM curves on the head and neck squamous cell carcinoma database, and P < 0.05 was significant. The division between high and low groups was chosen as the median for gene expression levels. The Kaplan–Meier Plotter database was constructed based on gene microarray and RNA-seq data from public databases such as GEO, EGA, and TCGA. It was used to integrate gene expression information and clinical prognostic values for meta-analysis and the study, discovery, and validation of survival-related molecular markers.
To analyze the potential functions and pathways of Radix Astragali acting on hypopharyngeal carcinoma, we used R software (R 4.2.0) to perform GO and KEGG enrichment analysis of potential targets of Radix Astragali for hypopharyngeal carcinoma, illustrating GO enrichment in terms of biological processes (BP), cellular components (CC), and molecular functions (MF). R-package-Bioconductor Cluster Profiler is an R package (R x64 4.0.3) widely used for gene bioinformatics analysis.
Autodock software is one of the software used for molecular docking. In this experiment, both Autodock 4 and Autodock Vina software were used for molecular docking, and Pymol software was used for molecular processing and visualization of docking results. The hub targets whose differential expression impacts the survival of head and neck squamous cell carcinoma patients and the corresponding active ingredients were used as molecular docking. 3D structures of the targets were obtained from the PDB database, and 3D structures of the active ingredients were obtained from the PubChm database. Autodock 4 and Pymol software were used to pre-process the target proteins, and Autodock Vina software was used for batch molecular docking. The technical roadmap of the entire study is shown in Fig. 1.
Network pharmacological study of Radix Astragali for treating hypopharyngeal carcinoma schematic diagram.
Because we use public and anonymous data, according to the ethics guidelines, neither informed consent nor approval of the ethics committee is required.
The active ingredients of Radix Astragali were searched in the TCMSP database using the keyword “Radix Astragali”, the active ingredients of Radix Astragali were screened using the screening criteria of OB > 30% and DL > 0.08. We finally obtained 20 active ingredients of Radix Astragali (Table 1).
We obtained 207 Radix Astragali-related targets from the TCMSP database, 271 Radix Astragali-related targets from the ETCM database, and 1484 Radix Astragali-related targets from the Symmap database. The above targets were integrated and de-duplicated to obtain 1589 Radix Astragali-related targets as the final Radix Astragali-related targets for further processing.
We used the keyword “Hypopharyngeal Carcinoma” in the Genecard database of hypopharyngeal carcinoma-related targets. We obtained 1050 hypopharyngeal carcinoma-related targets, and the targets obtained above were regarded as hypopharyngeal carcinoma-related targets.
We made a Venn diagram with Radix Astragali-related and hypopharyngeal carcinoma-related targets (Fig. 2). The intersection of the two was the target related to Radix Astragali for hypopharyngeal cancer, and a total of 109 potential targets of Radix Astragali for hypopharyngeal cancer were obtained. The potential targets of Radix Astragali for hypopharyngeal carcinoma were imported into the String database to construct a PPI network and imported into Cytoscape software for processing and analysis (Fig. 3), with Degree ≥ 10, Betweenness Centrality ≥ 0.00399091 and Closeness Centrality ≥ 0.391509434 as the screening criteria for screening hub targets to obtain hub targets, and a total of 20 hub targets were obtained (Table 2).
The Venn diagram about the target of Radix Astragali and the target of hypopharyngeal carcinoma. The blue circle represents the target of Radix Astragali, and the yellow circle represents the target of hypopharyngeal carcinoma. The intersection of the two circles represents the target of Radix Astragali for hypopharyngeal carcinoma.
PPI network of Radix Astragali in the treatment of hypopharyngeal carcinoma. The nodes represent potential therapeutic targets of Radix Astragali against hypopharyngeal carcinoma. The larger the node, the higher the corresponding target degree and the more connections to other nodes.
The hub targets were imported separately into The Kaplan–Meier Plotter online platform, and KM curves were plotted. The results showed that differential expression of a total of 17 hub genes had impacted the overall survival of patients with head and neck squamous cell carcinoma (Fig. 4). Targets that were genetically upregulated to improve overall survival in patients with head and neck squamous cell carcinoma included Interleukin-2 (IL2), Interleukin-1 (IL10), Apoptosis regulator Bcl-2 (BCL2), Tumor suppressor ARF (CDKN2A), C-X-C chemokine receptor type 4 (CXCR4), non-specific protein-tyrosine kinase (PTK2B), and Tumor necrosis factor (TNF); targets that were able to improve patient overall survival when gene expression was downregulated were non-specific protein-tyrosine kinase (AKT1), G1/S-specific cyclin-D1 (CCND1), C-X-C chemokine receptor type 8 (CXCL8), Epidermal growth factor receptor (EGFR), Cip1-interacting zinc finger protein (CDKN1A), Baculoviral IAP repeat-containing protein 5 (BIRC5), Glyceraldehyde-3-phosphate dehydrogenase (GAPDH), Hepatocyte growth factor receptor (MET), Myc proto-oncogene protein (MYC), and Transforming growth factor beta-1 proprotein (TGFB1).
The Kaplan–Meier curves. Prolonged median survival in patients with hypopharyngeal cancer when (A) (AKT1), (B) (CCND1), (C) (CXCL8), (D) (EGFR), (E) (TGFB1), (F) (MYC), (G) (CDKN1A), (H) (BIRC5), (I) (GAPDH), and (J) (MET) is overexpressed or (K) (BCL2), (L) (CDKN2A), (M) (IL2), (O) (IL10), (P) (TNF), and (Q) (CXCR4) expression is decreased.
To investigate the potential function of Radix Astragali for hypopharyngeal carcinoma, we subjected the potential targets of Radix Astragali for hypopharyngeal carcinoma to GO enrichment analysis, and we show the results of GO enrichment analysis in terms of BP, CC, and MF. P-values are arranged from most minor to most significant, and the top 10 BP, CC, and MF are shown in Figs. 5A,B, 6A,B and 7A,B. Figures 5B, 6B and 7B highlight the genes and relationship between functions. To explore the potential pathways of Radix Astragali for hypopharyngeal carcinoma, we subjected the potential targets of Radix Astragali for hypopharyngeal carcinoma to KEGG enrichment analysis, with the P-values arranged from smallest to largest, and the top 30 results of the enrichment results are displayed in Fig. 8.
Top ten significant biological process (BP) entries. (A) GO enrichment analysis of therapeutic targets for biological process. (B) Relationship between the therapeutic targets and biological process.
Top ten significant cell component (CC) entries. (A) GO enrichment analysis of therapeutic targets for cell components. (B) Relationship between the therapeutic targets and cell component.
Top ten significant molecular function (MF) entries. (A) GO enrichment analysis of therapeutic targets for molecular function. (B) Relationship between the therapeutic targets and molecular function.
KEGG enrichment analysis for therapeutic targets.
To simulate the process of mutual binding between the hub target whose differential expression of the target has an impact on the survival rate of head and neck squamous cell carcinoma patients, and the corresponding active ingredient of Radix Astragali, we made a molecular docking, and the docking with a release free energy < − 7 kcal/mol indicated that the complementary active ingredient and the target and bind effectively in the natural state. Based on the docking results, we plotted the heat map (Fig. 9), and we visualized the docking results for the docking with a release free energy < − 7 kcal/mol (Fig. 10) and the basic information of the docking results is displayed in Table 3.
Heatmaps of the docking scores of hub targets combined with the corresponding bioactive compound of Radix Astragali. The darker the blue, the more free energy the bioactive ingredient has to bind to the hub targets.
The significant molecular docking. (A) (CCND1, quercetin, − 9.2 kcal/mol); (B) (GAPDH, quercetin, − 9.2 kcal/mol); (C) (EGFR, kaempferol, − 9 kcal/mol); (D) (EGFR, quercetin, − 8.8 kcal/mol); (E) (GAPDH, kaempferol, − 8.8 kcal/mol); (F) (CXCR4, kaempferol, − 8.3 kcal/mol), (G) (CXCR4, quercetin, − 8.1 kcal/mol); (H) (CDKN2A, quercetin, − 8 kcal/mol), (I) (GAPDH, bifendate, − 7.9 kcal/mol); (J) (BIRC5, quercetin, − 7.6 kcal/mol), (K) (CDKN2A, kaempferol, − 7.6 kcal/mol); (L) (EGFR, bifendate, − 7.4 kcal/mol), (M) (BCL2, kaempferol, − 7.3 kcal/mol); (N) (CXCR4, bifendate, − 7.3 kcal/mol); (O) (IL2, quercetin, − 7.3 kcal/mol); (P) (MYC, quercetin, − 7.3 kcal/mol); (Q) (BCL2, quercetin, − 7.2 kcal/mol); (R) (CCND1, kaempferol, − 7.2 kcal/mol); (S) (MYC, kaempferol, − 7.2 kcal/mol); (T) (BIRC5, kaempferol, − 7.1 kcal/mol); (U) (IL2, kaempferol, − 7.1 kcal/mol); (V) (PTK2B, kaempferol, − 7.1 kcal/mol); (W) (PTK2B, quercetin, − 7.1 kcal/mol).
Hypopharyngeal carcinoma is a head and neck tumor, accounting for about 3% of head and neck tumors29. Hypopharyngeal carcinoma is highly malignant and has a poor healing process. Patients are often found to have reached an advanced stage and distant metastasis, with an overall survival rate of 30–35%1,2. Although people are looking for new treatments to improve the outcome of hypopharyngeal carcinoma, the outcome of hypopharyngeal carcinoma has yet to be greatly improved in recent years3,30, and new treatment strategies are urgently needed to improve the overall survival rate of hypopharyngeal carcinoma patients. Radix Astragali is one of the traditional Chinese medicines, and its various active ingredients have been proven to affect the biological behavior of tumors, such as proliferation, apoptosis, migration, and infiltration. Still, its effect on hypopharyngeal cancer and its mechanism has not been reported in the literature15,16,17. For these reasons, this study investigated the effect of Radix Astragali on hypopharyngeal carcinoma and the mechanism using network pharmacology and molecular docking techniques. In this study, we obtained Radix Astragalis and hypopharyngeal carcinoma-related targets from several databases. We intersected the above two targets, and the intersected targets were used as potential therapeutic targets of Radix Astragali for hypopharyngeal carcinoma for constructing a PPI network, GO, and KEGG enrichment analysis. The pivotal targets were obtained by PPI network analysis, and the KM curves of the pivotal targets were constructed to screen 17 pivotal targets that could influence the overall survival rate of hypopharyngeal carcinoma. Among them, the hub targets whose up-regulated expression could improve the overall survival rate of hypopharyngeal cancer patients included IL2, IL10, BCL2, CDKN2A, CXCR4, PTK2B, and TNF; the hub targets whose down-regulated expression could improve the overall survival rate of hypopharyngeal cancer patients included AKT1, CCND1, CXCL8, EGFR, CDKN1A, BIRC5, GAPDH, MET, MYC, and TGFB1. KEGG results showed that Radix Astragali acted on hypopharyngeal carcinoma through multiple signaling pathways, such as PI3K-Akt signaling pathway, IL-17 signaling pathway, TNF signaling pathway, and PD-L1 expression and PD-1 checkpoint pathway in cancer. These signaling pathways are well documented to affect the biological behavior of various tumors, such as the PI3K-Akt signaling pathway, which has been documented to promote apoptosis, inhibit tumor cell proliferation, and migrate a variety of cells. These signaling pathways have been extensively studied to influence the biological behavior of various tumors. For example, the PI3K-Akt signaling pathway has been shown in the literature to promote apoptosis, inhibit tumor cell proliferation, and migrate in a variety of cells31,32,33,34, The IL-17 signaling pathway mediates tumor growth35,36, and the TNF signaling pathway inhibits tumor metastasis when activated37,38, etc. Meanwhile, the KEGG results showed that the hub targets are involved in signaling multiple signaling pathways, such as AKT1 in the PI3K-Akt signaling pathway, TNF signaling pathway, PD-L1 expression, and PD-1 checkpoint pathway in cancer. For example, AKT1 is involved in the PI3K-Akt signaling pathway, TNF signaling pathway, PD-L1 expression, and PD-1 checkpoint pathway in cancer, TNF is involved in the L-17 signaling pathway, TNF signaling pathway, etc. By signaling multiple signaling pathways, the hub target connects multiple signaling pathways into an interoperable signaling network. Molecular docking results showed that quercetin and other active ingredients could freely bind to the hub targets, affecting the overall survival of hypopharyngeal carcinoma patients, with quercetin effectively docking with the most hub targets and Radix Astragali acting as the most promising target for hypopharyngeal carcinoma to improve the overall survival of patients. Although few studies have been conducted on the clinical use of Radix Astragali in the treatment of hypopharyngeal cancer, they have shown that Radix Astragali is one of the most effective herbs for reducing mortality in patients with advanced breast cancer, that Radix Astragali compounds are more effective in the treatment of hepatitis than controls; and that the combination of Radix Astragali and glucocorticoids (GCs) not only reduces the dosage of GCs but also mitigates the side effects caused by GCs. Quercetin is the main representative of the flavonol flavonoid subclass, and its anticancer effects have been demonstrated in various cancers. Quercetin inhibited the proliferation of colon, ovarian, and breast cancer cell lines39,40. Quercetin can also inhibit breast cancer progression through Akt-mTOR pathway-mediated autophagy-induced inhibition of cell migration and glycolysis41. Similar mechanisms have been found in quercetin-activated autophagy against gastric carcinogenesis42. Quercetin also has anticancer potential in head and neck squamous cell carcinoma. Studies have shown that quercetin inhibits the migration and invasion of head and neck squamous cell carcinoma cells by suppressing the expression of MMP-2 and MMP-943. Quercetin increases the expression levels of cytochrome c, apoptosis-inducing factor, and nucleic acid endonuclease G, thereby promoting apoptosis in oral cancer cells44. Quercetin also inhibits the growth of oral squamous cell carcinoma xenograft tumors by inducing miR-22 expression and inhibiting the WNT1/β-linker pathway in vivo45. Quercetin induced apoptosis in laryngeal cancer cells by inhibiting Akt/PKB phosphorylation and significantly decreasing anti-apoptotic Bcl-2 and Bcl-XL, thus acting as a chemosensitizer to cisplatin. Compared with cisplatin alone, quercetin combined with cisplatin increased the apoptosis rate from 18.7 to 42.2% in the laryngeal cancer HEP-2 cell line46. Therefore, combining previous studies with our results, quercetin may inhibit the migration and invasion of hypopharyngeal squamous cell carcinoma cells by regulating the PI3K-Akt signaling pathway, thus prolonging the overall survival of patients with hypopharyngeal carcinoma, which is also one of the directions of our subsequent studies.
We discovered Radix Astragali’s regulatory effect on hypopharyngeal carcinoma through pharmacological and molecular docking techniques. We are the first to find the above results. As we all know, network pharmacology is an efficient tool for the investigation of drug mechanisms. However, it is undeniable that the present study still has some limitations: firstly, In vitro and in vivo experiments were not conducted in this study, and the effect of Radix Astragali on hypopharyngeal carcinoma in the in vitro and in vivo situations and how quercetin binds to and modulates central targets were not explored; which we will continue to study in the next step; secondly, each database has a different focus, and there are differences between databases; If conditions permit, we will further employ Molecular quantification techniques (such as mass spectrometry) to confirm Quercetin’s presence to ensure the accuracy of the experimental results; therefore, there may be potential risks in the joint analysis of multiple databases. Thus, the discovery of new targets and pathways still needs to be done through basic laboratory experiments.
Through network pharmacology and molecular docking techniques, we found that the active ingredients of Radix Astragali act on multiple hub targets to regulate various signaling pathways connected to the hub targets to form a signaling network, through which Radix Astragali modulates the biological behavior of hypopharyngeal carcinoma and improves the overall survival rate of hypopharyngeal carcinoma patients. This process may be responsible for the inhibition of migration and invasion of hypopharyngeal squamous cell carcinoma cells by quercetin through the regulation of the PI3K-Akt signaling pathway. Among the active ingredients of Radix Astragali, quercetin has the most potential to become a therapeutic agent for hypopharyngeal carcinoma and to improve the overall survival rate of hypopharyngeal carcinoma patients.
The original contributions presented in the study are included in the article Material. Further inquiries can be directed to the corresponding authors.
Kyoto Encyclopedia of Genes and Genomes
Cip1-interacting zinc finger protein
Transforming growth factor beta-1 proprotein
Zhu, J. et al. Undifferentiated small round cell sarcoma of the postcricoid region of the hypopharynx: A rare case report and review of the literature. Onco Targets Ther. 14, 4537–4544 (2021).
Article PubMed PubMed Central Google Scholar
Zhang, M. et al. GPR12 inhibits migration and promotes apoptosis in esophageal cancer and hypopharyngeal cancer cells. Thorac. Cancer 12(10), 1525–1535 (2021).
Article CAS PubMed PubMed Central Google Scholar
Trang, N. T. K. & Yoo, H. Antitumor effects of valdecoxib on hypopharyngeal squamous carcinoma cells. Korean J. Physiol. Pharmacol. 26(6), 439–446 (2022).
Article CAS PubMed PubMed Central Google Scholar
Shimakage, M. et al. Expression of Epstein–Barr virus in mesopharyngeal and hypopharyngeal carcinomas. Hum. Pathol. 30(9), 1071–1076 (1999).
Article CAS PubMed Google Scholar
Gupta, T. et al. Squamous cell carcinoma of the hypopharynx: Single-institution outcome analysis of a large cohort of patients treated with primary non-surgical approaches. Acta Oncol. 48(4), 541–548 (2009).
Yu, Z. et al. Optimization and evaluation of Radix Astragali polysaccharide injectable thermoresponsive in-situ gels. PLoS ONE 12(3), e0173949 (2017).
Article PubMed PubMed Central Google Scholar
Zhang, X., Qiu, H., Li, C., Cai, P. & Qi, F. The positive role of traditional Chinese medicine as an adjunctive therapy for cancer. Biosci. Trends 15(5), 283–298 (2021).
Article CAS PubMed Google Scholar
Ge, H. et al. Traditional Chinese medicines as effective reversals of epithelial-mesenchymal transition induced-metastasis of colorectal cancer: Molecular targets and mechanisms. Front. Pharmacol. 13, 842295 (2022).
Article CAS PubMed PubMed Central Google Scholar
Jiang, H. et al. Traditional Chinese medicine for adjuvant treatment of breast cancer: Taohong Siwu decoction. Chin. Med. 16(1), 129 (2021).
Article CAS PubMed PubMed Central Google Scholar
Zhao, Y. Z., Dai, Y. Z. & Nie, K. Research progress on the antiemetic effect of traditional Chinese medicine against chemotherapy-induced nausea and vomiting: A review. Front. Pharmacol. 12, 790784 (2021).
Article ADS CAS PubMed Google Scholar
Szabo, N. J. Dietary safety of cycloastragenol from Radix Astragali spp.: Subchronic toxicity and genotoxicity studies. Food Chem. Toxicol. 64, 322–334 (2014).
Article CAS PubMed Google Scholar
Song, J. et al. Safety evaluation of Radix Astragali extract mixture HT042 and its constituent herbs in Sprague–Dawley rats. Phytomedicine 32, 59–67 (2017).
Article CAS PubMed Google Scholar
Sun Meng, Wang Jingjing, Shi Zhu, Wang Shuanjin. Effects of apatinib mesylate combined with astragaloside IV on the proliferation, migration and invasion of primary liver cancer cells%J Advances in Anatomical Science. 29(01), 55–59 (2023 ).
Luan Lina, Zhu Peiqin, He Zhu, et al. Animal experiments on astragaloside IV alleviating epirubicin-related cardiotoxicity%J Chinese Clinical Medicine. 29(06), 1006–1011 (2022).
Lou, Y. et al. Astragali Radix and its main bioactive compounds activate the Nrf2-mediated signaling pathway to induce P-glycoprotein and breast cancer resistance protein. J. Ethnopharmacol. 228, 82–91 (2019).
Article CAS PubMed Google Scholar
Wu, J., Xu, H., Zhang, L. & Zhang, X. Radix Astragali and tanshinone help carboplatin inhibit B16 tumor cell growth. Technol. Cancer Res. Treat. 15(4), 583–588 (2016).
Article CAS PubMed Google Scholar
Wang, Y. et al. Anticancer effect of Radix Astragali on cholangiocarcinoma in vitro and its mechanism via network pharmacology. Med. Sci. Monit. 26, e921162 (2020).
CAS PubMed PubMed Central Google Scholar
Na, D., Liu, F. N., Miao, Z. F., Du, Z. M. & Xu, H. M. Radix Astragali extract inhibits destruction of gastric cancer cells to mesothelial cells by anti-apoptosis. World J. Gastroenterol. 15(5), 570–577 (2009).
Article CAS PubMed PubMed Central Google Scholar
Zhang, Y. M. et al. The effects of Radix Astragali polysaccharide on bone marrow-derived mesenchymal stem cell proliferation and morphology induced by A549 lung cancer cells. Med. Sci. Monit. 25, 4110–4121 (2019).
Article CAS PubMed PubMed Central Google Scholar
Guo, H. et al. Radix Astragali saponins inhibit cell growth, aerobic glycolysis and attenuate the inflammatory response in a DSS-induced colitis model. Int. J. Mol. Med. 43(2), 1041–1048 (2019).
Song, Y., Yang, J., Bai, W. L. & Ji, W. Y. Antitumor and immunoregulatory effects of Radix Astragali on nasopharyngeal carcinoma in vivo and in vitro. Phytother. Res. 25(6), 909–915 (2011).
Article CAS PubMed Google Scholar
Zhou, Z., Meng, M. & Ni, H. Chemosensitizing effect of Radix Astragali polysaccharides on nasopharyngeal carcinoma cells by inducing apoptosis and modulating expression of Bax/Bcl-2 ratio and caspases. Med. Sci. Monit. 23, 462–469 (2017).
Article CAS PubMed PubMed Central Google Scholar
Zhou, Z. et al. Applications of network pharmacology in traditional Chinese medicine research. Evid. Based Complement. Altern. Med. 2020, 1646905 (2020).
Luo, T. T. et al. Network pharmacology in research of Chinese medicine formula: Methodology, application and prospective. Chin. J. Integr. Med. 26(1), 72–80 (2020).
Article CAS PubMed Google Scholar
Zhang, R., Zhu, X., Bai, H. & Ning, K. Network pharmacology databases for traditional Chinese medicine: Review and assessment. Front. Pharmacol. 10, 123 (2019).
Article PubMed PubMed Central Google Scholar
Liu, J. et al. Network pharmacology prediction and molecular docking-based strategy to discover the potential pharmacological mechanism of Huai Hua San against ulcerative colitis. Drug Des. Dev. Ther. 15, 3255–3276 (2021).
Xu, X. et al. A novel chemometric method for the prediction of human oral bioavailability. Int. J. Mol. Sci. 13(6), 6964–6982 (2012).
Article CAS PubMed PubMed Central Google Scholar
Tao, W. et al. Network pharmacology-based prediction of the active ingredients and potential targets of Chinese herbal Radix Curcumae formula for application to cardiovascular disease. J. Ethnopharmacol. 145(1), 1–10 (2013).
Article CAS PubMed Google Scholar
Chen, Y. et al. Stathmin1 overexpression in hypopharyngeal squamous cell carcinoma: A new promoter in FaDu cell proliferation and migration. Int. J. Oncol. 50(1), 31–40 (2017).
Zeng, Y. L. et al. Diagnostic and prognostic value of lncRNA cancer susceptibility candidate 9 in hepatocellular carcinoma. World J. Gastroenterol. 25(48), 6902–6915 (2019).
Article CAS PubMed PubMed Central Google Scholar
Kumar, A. et al. Targeting Akt/NF-kappaB/p53 pathway and apoptosis inducing potential of 1,2-benzenedicarboxylic acid, bis (2-methyl propyl) ester isolated from onosma bracteata wall, against human osteosarcoma (MG-63) cells. Molecules 27(11), 3478 (2022).
Article CAS PubMed PubMed Central Google Scholar
Chen, H. et al. CHRDL2 promotes osteosarcoma cell proliferation and metastasis through the BMP-9/PI3K/AKT pathway. Cell Biol. Int. 45(3), 623–632 (2021).
Article CAS PubMed PubMed Central Google Scholar
Zhou, L., Yu, Y., Sun, S., Zhang, T. & Wang, M. Cry 1 regulates the clock gene network and promotes proliferation and migration via the Akt/P53/P21 pathway in human osteosarcoma cells. J. Cancer 9(14), 2480–2491 (2018).
Article PubMed PubMed Central Google Scholar
Liu, J., Chen, M., Ma, L., Dang, X. & Du, G. LncRNA GAS5 suppresses the proliferation and invasion of osteosarcoma cells via the miR-23a-3p/PTEN/PI3K/AKT pathway. Cell Transplant. 29, 963689720953093 (2020).
Wang, X. et al. Inflammatory cytokines IL-17 and TNF-alpha up-regulate PD-L1 expression in human prostate and colon cancer cells. Immunol. Lett. 184, 7–14 (2017).
Article CAS PubMed PubMed Central Google Scholar
Grivennikov, S. I. et al. Adenoma-linked barrier defects and microbial products drive IL-23/IL-17-mediated tumor growth. Nature 491(7423), 254–258 (2012).
Article ADS CAS PubMed PubMed Central Google Scholar
Horssen, R. V., Hagen, T. L. M. T. & Eggermont, A. M. M. TNF-α in cancer treatment: Molecular insights, antitumor effects, and clinical utility. Oncol. Sarcomas 11, 397–408 (2006).
Wu, Y. & Zhou, B. P. TNF-alpha/NF-kappaB/snail pathway in cancer cell migration and invasion. Br. J. Cancer 102(4), 639–644 (2010).
Article CAS PubMed PubMed Central Google Scholar
Amorim, R. et al. Monocarboxylate transport inhibition potentiates the cytotoxic effect of 5-fluorouracil in colorectal cancer cells. Cancer Lett. 365(1), 68–78 (2015).
Article CAS PubMed Google Scholar
Xintaropoulou, C. et al. A comparative analysis of inhibitors of the glycolysis pathway in breast and ovarian cancer cell line models. Oncotarget 6(28), 25677–25695 (2015).
Article PubMed PubMed Central Google Scholar
Jia, L. et al. Quercetin suppresses the mobility of breast cancer by suppressing glycolysis through Akt-mTOR pathway mediated autophagy induction. Life Sci. 208, 123–130 (2018).
Article CAS PubMed Google Scholar
Wang, K. et al. Quercetin induces protective autophagy in gastric cancer cells: Involvement of Akt-mTOR- and hypoxia-induced factor 1alpha-mediated signaling. Autophagy 7(9), 966–978 (2011).
Article CAS PubMed Google Scholar
Chan, C. Y., Lien, C. H., Lee, M. F. & Huang, C. Y. Quercetin suppresses cellular migration and invasion in human head and neck squamous cell carcinoma (HNSCC). Biomedicine 6(3), 15 (2016).
Article PubMed PubMed Central Google Scholar
Ma, Y. S. et al. Quercetin induced apoptosis of human oral cancer SAS cells through mitochondria and endoplasmic reticulum mediated signaling pathways. Oncol. Lett. 15(6), 9663–9672 (2018).
PubMed PubMed Central Google Scholar
Zhang, C., Hao, Y., Sun, Y. & Liu, P. Quercetin suppresses the tumorigenesis of oral squamous cell carcinoma by regulating microRNA-22/WNT1/beta-catenin axis. J. Pharmacol. Sci. 140(2), 128–136 (2019).
Article CAS PubMed Google Scholar
Sharma, H., Sen, S. & Singh, N. Molecular pathways in the chemosensitization of cisplatin by quercetin in human head and neck cancer. Cancer Biol. Ther. 4(9), 949–955 (2005).
Article CAS PubMed Google Scholar
The authors are very grateful for the contributions of the TCMSP database, ETCMdatabase, symMap database, and Genecard database that provide information on cancer research, and all colleagues involved in the study.
The Liaoning Natural Science Foundation Program (No. 2019-ZD-0901).
Department of Otorhinolaryngology-Head and Neck Surgery, Central Hospital of Chaoyang, Liaoning, 122000, China
Jianing Zhang & Lianhe Li
You can also search for this author in PubMed Google Scholar
You can also search for this author in PubMed Google Scholar
(I) Conception and design: L.L.; (II) Administrative support: Central Hospital of Chaoyang; (III) Provision of study materials or patients: the Traditional Chinese Medicine Systems Pharmacology Database and Analysis Platform (TCMSP) database, The Encyclopedia of Traditional Chinese Medicine (ETCM) database, SymMap database, and the Genecard database; (IV) Collection and assembly of data: J.Z.; (V) Data analysis and interpretation: J.Z.; (VI) Manuscript writing: All authors; (VII) Final approval of manuscript: All authors.
The authors declare no competing interests.
Springer Nature remains neutral with regard to jurisdictional claims in published maps and institutional affiliations.
Open Access This article is licensed under a Creative Commons Attribution 4.0 International License, which permits use, sharing, adaptation, distribution and reproduction in any medium or format, as long as you give appropriate credit to the original author(s) and the source, provide a link to the Creative Commons licence, and indicate if changes were made. The images or other third party material in this article are included in the article's Creative Commons licence, unless indicated otherwise in a credit line to the material. If material is not included in the article's Creative Commons licence and your intended use is not permitted by statutory regulation or exceeds the permitted use, you will need to obtain permission directly from the copyright holder. To view a copy of this licence, visit http://creativecommons.org/licenses/by/4.0/.
Zhang, J., Li, L. Network pharmacology prediction and molecular docking-based strategy to explore the potential mechanism of Radix Astragali against hypopharyngeal carcinoma. Sci Rep 14, 516 (2024). https://doi.org/10.1038/s41598-023-50605-3
DOI: https://doi.org/10.1038/s41598-023-50605-3
Anyone you share the following link with will be able to read this content:
Sorry, a shareable link is not currently available for this article.
Provided by the Springer Nature SharedIt content-sharing initiative
By submitting a comment you agree to abide by our Terms and Community Guidelines. If you find something abusive or that does not comply with our terms or guidelines please flag it as inappropriate.
Scientific Reports (Sci Rep) ISSN 2045-2322 (online)
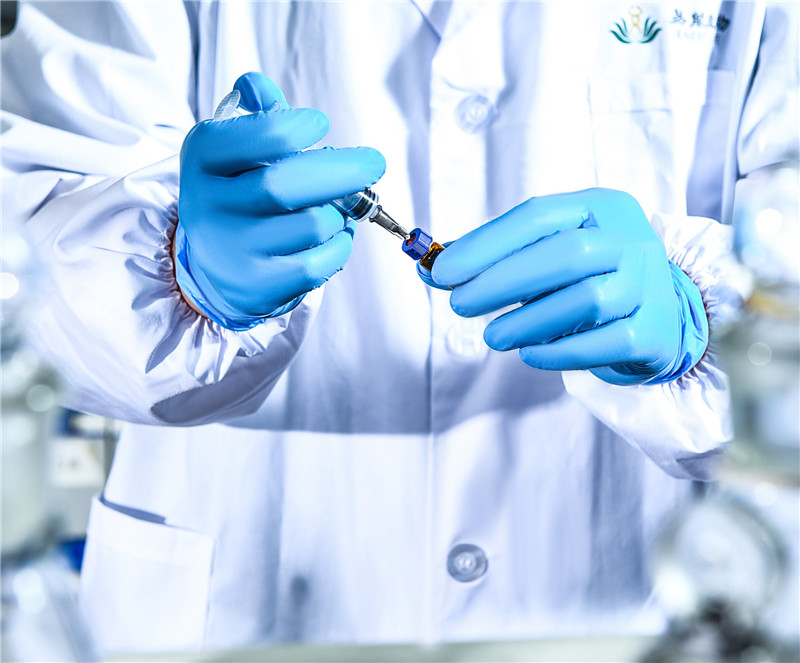
Natural Medicine Cro Sign up for the Nature Briefing: Cancer newsletter — what matters in cancer research, free to your inbox weekly.