ⓒAsia Today, unauthorized reproduction and redistribution prohibited
'Wage tug of war' Samsung Electronics proposes a basic increase rate of 2%...The north side... Expanded Ptfe Gaskets
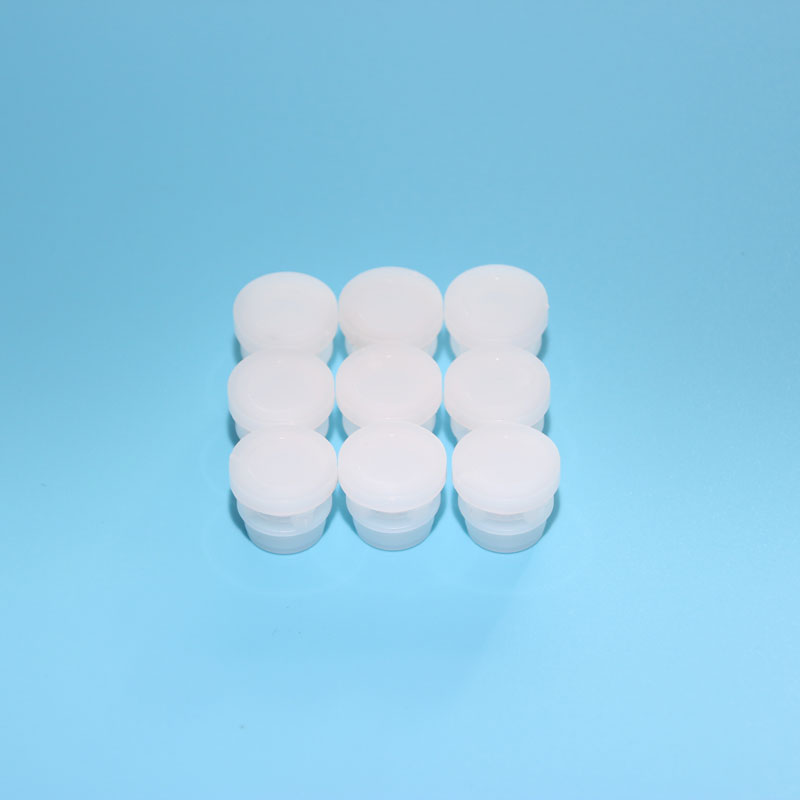
Osstem Implant, what about minority shareholders?
Confirmation of 63 suspected illegal activities such as tower crane sabotage...Ministry of Land, Infrastructure and Transport, Carl..
SK Hynix overcame the bad news of the Dalian plant in China acquired from Intel and launched a new...
[Photo] Lee Jae-myung confirming the sentence of the Constitutional Court ruling on 'Complete inspection'...
Prime Minister Han Deok-soo “25 trillion won for R&D such as semiconductor, bio, and aerospace..
Lee Chan-won, cabbage dish for Super Junior Eunhyuk at 'Pyeon Restaurant'..
The end of the long journey of the Sangwol Society Indian pilgrimage group... Joe among 30,000 people...
Lee Chan-won, cooking cabbage pancake at 'Pyeon Restaurant' "Reminds me of my grandmother..
Jogyesa Temple held the funeral ceremony for the Sangwol Gyeol Indian pilgrimage
Twice, the first K-pop girl group to sell out at Sophie Stadium in the U.S.
Sangwolgyeol Indian pilgrimage group heading to Jogyesa Temple
About Us |Advertising Guide |Subscribe |Report 24 hours |Grievance Handling |Membership Terms |Privacy Policy |Youth Protection Policy |Copyright Regulations |Recruitment |site map
Registration number: Seoul A00160 |Registration Date: January 18, 2006 |Title: Asia Today |Chairman: Injun Song |PublisherㆍEditor : Woo Jong-soon
Inyeong Building, 34 Uisadang-daero 1-gil, Yeongdeungpo-gu, Seoul |Publication Date: November 11, 2005 |Main phone number: 02) 769-5000 |Youth Protection Officer: Seong Hee-je
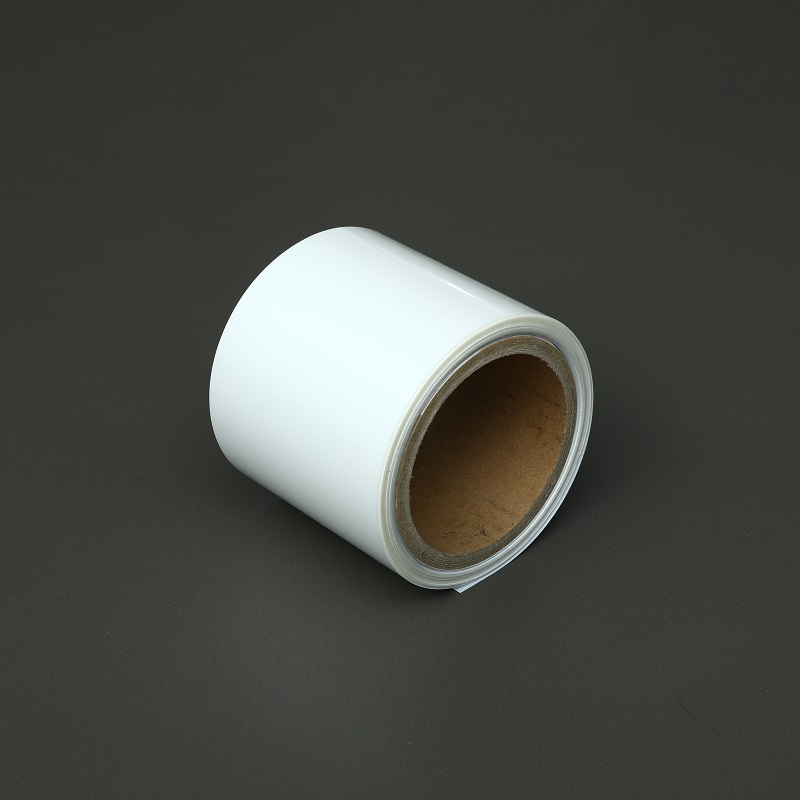
Membran Vent Asia Today complies with the Code of Ethics of the Internet Newspaper Commission.Copyright by ASIATODAY Co., Ltd.All Rights Reserved