This content is made possible by our sponsors. Click here to learn more.
When it comes to Roofing Nails- Maze Nails has you covered! “Nail it Once. Nail it Right.®” is the Maze motto. This means that contractors get the job done right the first time and don’t have to worry about spending time or money on callbacks. Maze uses the best steel possible and designs and manufactures the nails so that our LIFETIME WARRANTY really means something. Consider 30-year or 50-year shingles that get applied with 5 or 10 year import, electroplated nails. Homeowners are often horrified to see their expensive, top-notch shingles delivered with lousy nails. It just doesn’t make sense. Welded Panels
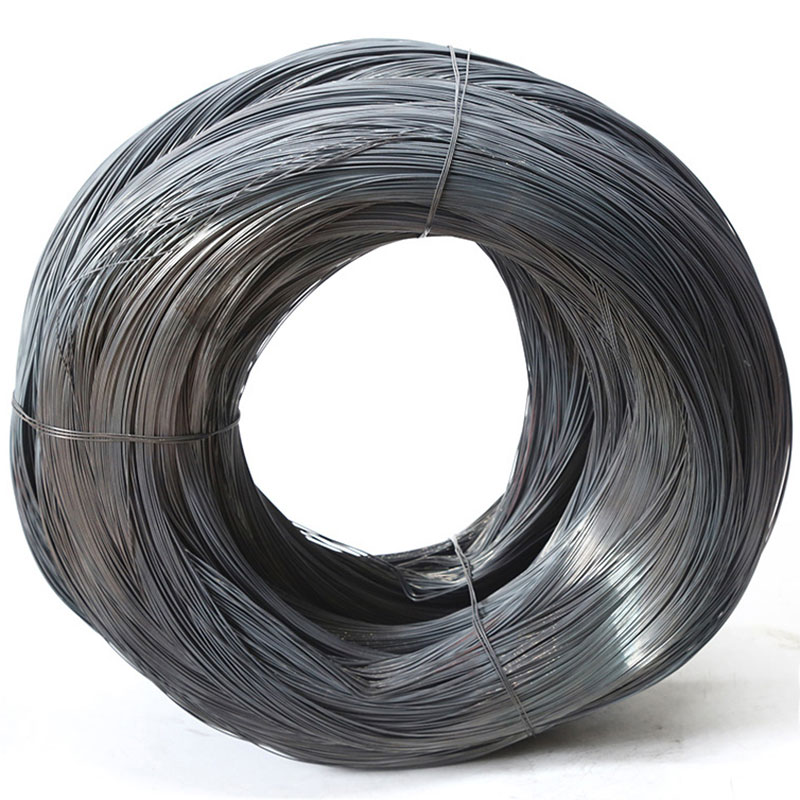
Maze Nails are designed for the secure application of asphalt and fiberglass shingles and cedar shingles and shakes. Ring and spiral shanks provide extra holding power, especially in high wind/hurricane areas. STORMGUARD® Double Hot-Dipped Zinc-Coating provides extra corrosion-resistance. Manufactured to meet ASTM specs. F1667 and ASTM A-153 for Hot-Dipped Zinc-coating. Maze offers a variety of sizes to meet all your recommended installation guidelines. Type 304 and 316 Stainless Steel Roofing Nails are also available for coastal applications. Due to popular demand, Maze has a full line of Coil-ated® Roofing nails designed to fit the popular roofing nailers. These roofing nails are 100% American Made which ensures the same top-quality nails everytime! Don’t let the nails be the weakest link in your roofing job—- look for the red and yellow MAZE NAILS boxes for your next roofing job.
LBM Journal is the leading media company serving lumberyards, building material distributors, wholesalers, manufacturers, and service providers.
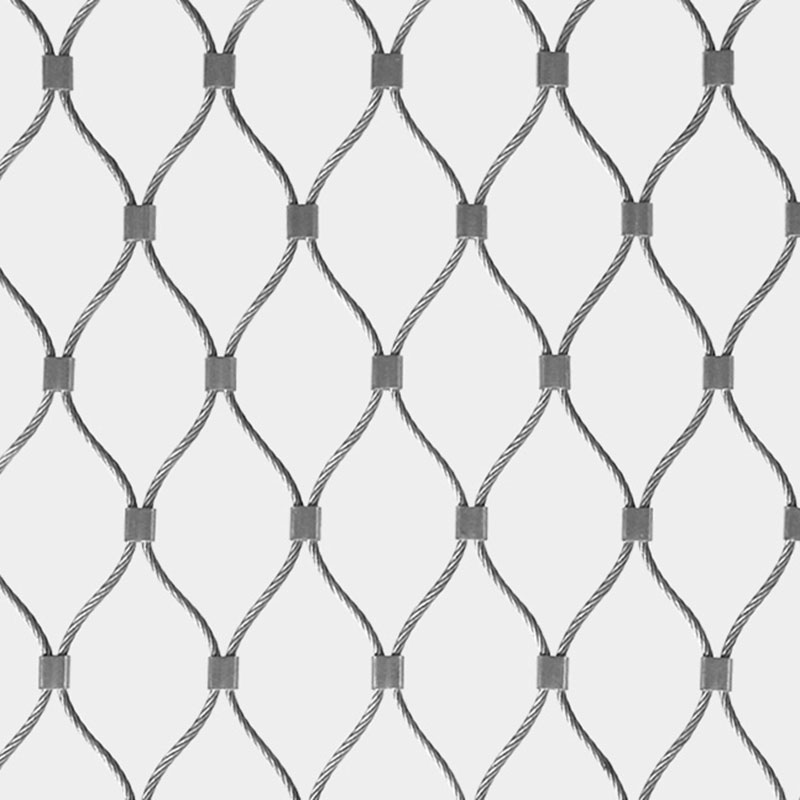
Welded Wire Mesh Panel Copyright © 2023 LBM Journal. All Rights Reserved.