Benign pigmented lesions are a common cosmetic concern for many patients with a history of moderate sun exposure or photodamage. Solar lentigines may be the only sign of aging, which may create a psychological burden to patients. Multiple treatment modalities are available, such as over-the-counter and prescription topical creams, and office-based procedures, including liquid nitrogen, chemical peels, and light-based modalities. Laser-based treatment for pigmented lesions is based on our understanding of selective photothermolysis by Anderson and Parrish. [1] This principle focuses on the lasers’ specificity to a wavelength that targets a dedicated chromophore, in this case melanin. Additionally, melanin absorption decreases as the wavelength increases, which allows safe laser use even in darker skin types. [2] Given the high energy required for laser treatment, collateral damage can be minimized through a targeted approach. Lasers continue to evolve, as does the associated nomenclature. Historically, lasers have been referred to based on their design, such as the Q-switched laser. For simplicity and in an effort to avoid confusion, this article generally refers to lasers based on their pulse duration and focuses on common laser-based treatment modalities. Basic settings and approaches to benign pigmented lesions are included. For more details on the history and science of lasers regarding the treatment of benign pigmented lesions, refer to Historical Science Overview.
Related articles include Laser Treatment of Scars and Stria Distensae, Laser Treatment of Acquired and Congenital Vascular Lesions, and Laser Eyelid Tissue Resurfacing. Alex Yag Laser Machine
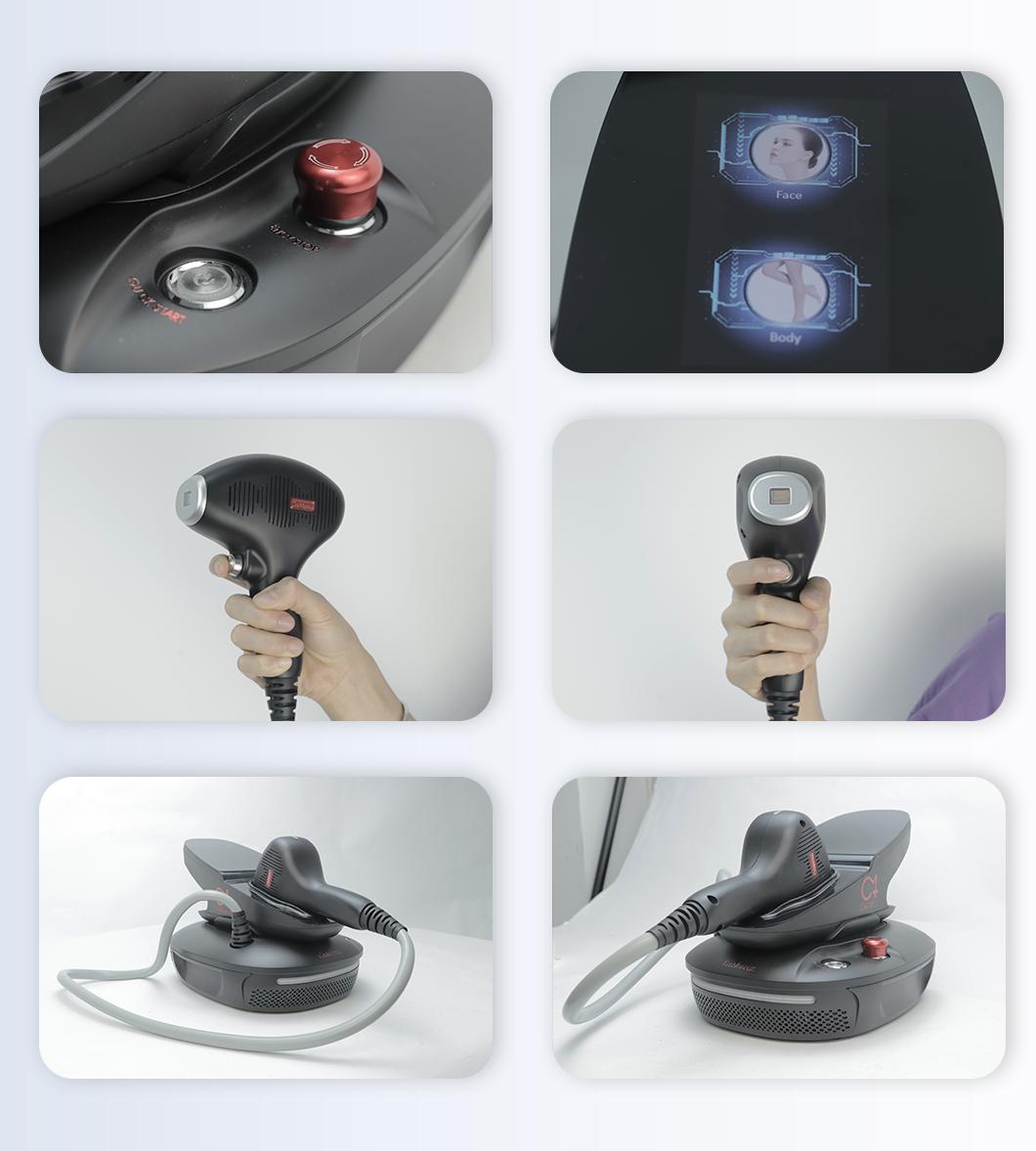
The cause of melanosomal destruction is unknown. Plasma formation probably does not occur. The peak powers produced with lasers used to interact with melanosomes are quite low for such an occurrence. More likely explanations are shockwave and/or cavitation damage, the photomechanical physical effects produced from thermal expansion, and/or the extreme temperature gradients created within the melanosome. Studies of acoustic waves generated by pulsed irradiation of melanosomes and pigmented cells support this possibility. Melanin absorbs and localizes the high-intensity irradiation from Q-switched lasers, thereby creating a sharp temperature gradient between the melanosome and its surrounding other structures. This gradient leads to thermal expansion and the generation and propagation of acoustic waves, which can mechanically damage the melanosome-laden cells.
Tissue repair following laser-induced melanosomal disruption demonstrates a 2-staged initial transient cutaneous depigmentation followed by subsequent repigmentation weeks later. Black guinea pig skin irradiated with 40-nanosecond Q-switched ruby laser (QSRL) pulses at a radiant exposure of 0.4 J/cm2 or greater whitens immediately, fades in 20 minutes, depigments 7-10 days later, and then repigments 4-8 weeks after treatment. The repigmented guinea pig skin displays a persistent leukotrichia, which can last up to 4 months after laser irradiation. Guinea pig skin exposed to a radiant exposure of less than that of the threshold exposure (< 0.3 J/cm2) undergoes paradoxical melanogenesis. This may be due to either a sublethal change in the melanosome (interfering with the normal feedback inhibition of melanogenesis) or simply postinflammatory hyperpigmentation. Further studies are required to evaluate the therapeutic implications of this paradoxical reaction.
Laser irradiation leads to histologic melanosomal disruption and vacuolization of pigment-laden cells in the basal layer. Both keratinocytes and melanocytes exhibit pigment and nuclear material condensation at the periphery of laser-irradiated cells. This leads to a characteristic "ring-cell" appearance. Epidermal necrosis and regeneration of a pigmented epidermis follow over the next 7 days.
Observations of the effects on human skin are similar to those of guinea pig skin. The QSRL targets melanosomes. Subsequently, sloughing of the killed pigmented cells occurs. Transient hypopigmentation is followed by gradual repigmentation to the normal constitutive color. Other short-pulsed, high-fluence specific pigmented lesion lasers produce similar clinical and histologic findings in human skin.
Three action-spectrum studies have analyzed the ability of different-wavelength pulsed lasers to disrupt cutaneous pigment. Anderson et al evaluated the effects of a Q-switched neodymium-doped yttrium aluminum garnet (Nd:YAG) laser with a pulse duration of 10-12 nanoseconds, at 3 distinct wavelengths (355, 532, and 1064 nm), on a guinea pig's skin. The threshold exposure for immediate skin whitening, the sign of laser-induced melanosomal changes, required an energy fluence of 0.11, 0.2, and 1 J/cm2 at 355, 532, and 1064 nm, respectively. These findings show that the threshold exposure dose is wavelength-dependent. Furthermore, longer wavelengths (which are less well-absorbed by melanin) require a higher energy fluence to induce these changes. At all evaluated wavelengths, electron microscopic examination revealed disrupted melanosomes within keratinocytes and melanocytes. Histologically, irradiated basal cells show a characteristic ring-cell appearance. This appearance is thought to be secondary to vacuolization and peripheral condensation of the cellular pigment. As expected, the transient, immediate whitening of the laser-treated area exhibits delayed epidermal depigmentation followed by repigmentation back to constitutive skin color.
Flashlamp-pulsed tunable lasers with a pulse duration of 750 nanoseconds also demonstrate the relationship between wavelength and whitening threshold. The threshold fluence was found to be 0.44, 0.62, 0.76, and 0.86 J/cm2 at 435, 488, 532, and 560 nm, respectively.
Sherwood et al performed an action-spectrum study of guinea pig skin using a flashlamp-pulsed tunable laser with a pulse duration of 300 nanoseconds at 5 different wavelengths (504, 590, 720, and 750 nm). They found the 504-nm wavelength produced the most pigment-specific injury because the longer wavelengths caused disruption of the basement membrane with pigmentary incontinence.
In current practice, numerous lasers can specifically target pigmented lesions, including red-light lasers (eg, 694-nm ruby, 755-nm alexandrite), green-light lasers (eg, 510-nm pulsed dye, [3, 4, 5, 6] 532-nm frequency-doubled Nd:YAG), and near-infrared lasers (eg, 1064 nm Nd:YAG). The wide range of lasers that can be used to treat pigment is a result of the broad absorption spectrum of melanin. Even so, other less pigment-specific lasers have been used to treat pigmented lesions, including the argon, krypton, copper, carbon dioxide, and, most recently, erbium-doped yttrium aluminium garnet laser (Er:YAG) lasers. Other lasers have been proposed for use, and their use with laser with cooling units either a cold tip or blowing cold air has increased the effectiveness of treatment. [7]
The carbon dioxide laser exerts its effect on tissue by simple vaporization of water-containing cells. Textural skin changes and scarring may result from this nonselective destruction. A very low-wattage carbon dioxide laser appears to reduce the risk of scarring and has been used effectively to treat superficial epidermal pigmented lesions, such as solar lentigines. The Er:YAG laser also vaporizes water-containing cells and may more precisely ablate superficial layers of skin compared with the carbon dioxide laser. Note that wavelengths not selectively absorbed by melanin indiscriminately destroy pigmented and nonpigmented structures in the skin.
Alternatively, lasers with wavelengths that are both (1) preferentially absorbed by melanin over other cutaneous chromophores (eg, hemoglobin) and (2) penetrate to the depth of the targeted pigment can be used to more selectively target cutaneous pigment. Lasers emitting wavelengths of 630-1100 nm may provide selective melanosome absorption, good skin penetration because of these longer wavelengths, and selection of melanin over hemoglobin.
Pulsed lasers with appropriate wavelengths have a distinct theoretical advantage over continuous-wave devices in the selective destruction of cutaneous pigment. The green and blue light (488 and 514 nm, respectively) of the argon laser is specifically absorbed by melanin. The problem with the system is that it functions as a continuous-wave laser. Thus, although this laser selectively targets the melanin chromophore, the heat produced dissipates from the absorbing melanosomes, causing thermal damage to surrounding tissue with resultant hypopigmentation and potential scarring. Similar findings can ensue after use of the krypton (520-530 nm) and copper (511 nm) lasers.
Pigment-specific lasers can be divided into 3 categories: (1) green, (2) red, and (3) near-infrared. Green-light lasers are further subdivided into both pulsed and nonpulsed systems. Red-light lasers are subdivided into short-pulsed (Q-switched) and long-pulsed (normal-mode) systems. The currently available near-infrared laser is short-pulsed (Q-switched). Green-light lasers do not penetrate as deeply into the skin as the red-light and near-infrared lasers, owing to their shorter wavelengths. Therefore, green-light lasers are effective only in the treatment of epidermal pigmented lesions, as demonstrated in the images below.
Schawlow and Townes were working with microwaves in 1958 when they first proposed a technique for the generation of monochromatic radiation by stimulated emission. They produced monochromatic radiation in the infrared optical region of the electromagnetic spectrum with an alkali vapor used as the active medium. In 1960, Maiman developed stimulated emission of a red-light beam with a wavelength of 694 nm using a ruby crystal. This was the first working laser and is the prototype from which today's lasers are derived.
Since 1960, research and technical advances have adapted lasers to dermatology. In 1963, Goldman first experimented with a normal-mode (500-microsecond pulse duration), 694-nm ruby laser pulse on human skin. The darker the skin color, the more the laser was absorbed. Based on these observations, he speculated that melanin selectively absorbs laser light.
In later studies, Goldman used a QSRL (50-microsecond pulse duration) and found that the damage threshold of pigmented lesions was independent of skin color. This suggested a more selective effect, perhaps at the level of the melanosome. The early work with the ruby laser consisted of ablation techniques. Little bleeding was noted after nonspecific damage to the superficial dermal layers. Small areas of skin could be treated with high-intensity radiation with few complications.
Approximately 20 years later, Polla et al [8] and Dover et al, [9] in separate studies, demonstrated that the QSRL targeted individual melanosomes. Electron microscopic analysis of these thermally damaged targeted melanosomes revealed membrane disruption and disorganization of the internal contents. The destruction of melanosomes is pulse-width-dependent; pulse durations of 40 nanoseconds and 750 nanoseconds both disrupt melanosomes, but longer pulse durations (eg, 400 microseconds) do not damage the melanosomes. This is consistent with the theory of selective photothermolysis, which states that the pulse duration of an emitted laser wavelength must be less than the thermal relaxation time of the targeted object. A typical 1-µm melanosome has a thermal relaxation time of 0.5-1 microseconds.
From 2001-2006, most investigators have studied and reported good effects with Q-switched lasers. Q-switched lasers are considered by some authorities to be the lasers of choice for pigmented melanocytic processes. This has been underlined by reports that Q-switched laser is a treatment of choice for the nevus of Ota. [10] Fraxel laser may be an option for pigmented lesions and pigmentation itself. [11]
The newest treatment for benign pigmented lesions involves the use of the picosecond 755-nm alexandrite laser. [12] The picosecond laser has found its greatest utility for tattoos. [13, 14]
Epidermal pigmented lesions have pigment isolated above the basement membrane. Any treatment that targets the epidermis can be used for treatment of these lesions. Many topical lotions, chemical peels, and light-based treatments have been developed for the treatment of pigmented epidermal lesions. Sun protection should be emphasized as the cornerstone to any treatment regimen. Most commonly, the treatment of solar lentigines begins with topical bleaching creams alone or in combination agents such as hydroquinone, tretinoin, or low-potency steroids. Liquid nitrogen is often used as the first office-based treatment for solar lentigines and seborrheic keratosis. These approaches are less specific than currently available lasers.
The best treatment for photo-related changes begins with prevention. Results of prolonged sun exposure can manifest as solar lentigines in addition to other changes of tissue texture [15] and laxity, not to mention the development of precancerous or cancerous pathology. The pigment changes of photoaging occur in more than 90% of patients older than 60 years. [16, 17] Additionally, pigment-related changes have been reported as a major concern for aging, in particular patients of Asian descent, which may be the only sign of aging. [2, 17] Patients often initiate treatment on their own from information found on the Internet or based on advice from family and friends. Many over-the-counter treatments are available for the treatment of pigmentary changes and photoaging. As these products may not produce the desired results, patients may seek expert consultation from their provider.
Patient screening is essential to any treatment success. For example, gold is an older treatment approach for connective-tissue diseases such as rheumatoid arthritis. Any patient who received treatment with gold is contraindicated for laser treatment, given the paradoxical hyperpigmentation that may occur. [2, 18] A more common pretreatment consideration is the patient’s potential to develop postinflammatory pigment alteration (PIPA). Patients with a history of PIPA or those with a darker complexion should be considered for prophylaxis treatment with hydroquinone. Treatment may begin 3-4 weeks before the procedure and should be discontinued 1 week prior to laser treatment. These patients should resume prophylaxis treatment immediately post laser therapy. Hydroquinone for 2-4 weeks or a mild-potency steroid used for 3-4 days is a common posttreatment prophylaxis. In the authors’ practice, PIPA prophylaxis is completed with a single office-based application of clobetasol applied to the treated area. Lastly regarding prophylaxis, patients with a history of oral herpetic infection should be prophylactically covered with antivirals when treating lesions on the face. [2, 18]
Clinical evaluation should be conservative when selecting appropriate lesions to treat. Rogers et al note 90% of malignant lesions demonstrate dermoscopic change within 3 months. [19] Any suspicious lesion should undergo biopsy before treating for cosmetic improvement. Further, Rogers et al suggest caution if treating pigmented lesions, in particular nevi, in patients with greater than 50 nevi, given the increased risk of melanoma. [19] Hibler et al note that 7.4% of cases of lentigo maligna had a prior cosmetic procedure, with 29.7% receiving laser-based therapies. [20] To further emphasize the significance of patient screening, a case report by Cohen et al reports desmoplastic melanoma arising after a lentigo was treated with a 1064-nm laser. [16] For these reasons, the authors do not recommend laser treatment for congenital or acquired melanocytic nevi.
When lasers are selected as the treatment for epidermal lesions, nanosecond lasers have been the criterion standard. While picosecond lasers are emerging as a safe and effective treatment for pigmented lesions, [21] select long-pulse lasers still contribute. The pulse duration for long-pulsed lasers are typically in milliseconds, making nanosecond lasers one million times faster. Faster still, picosecond lasers have the shortest pulse duration of currently available lasers. Pulse durations in the nanosecond range make these lasers effective at targeting the microscopic structure of the melanosome. Therapeutic effects are derived from the photoacoustic damage of melanosomes. [15] Melanosomes are 0.7 µm in diameter in types I and II skin and 1 µm or more in diameter in darker skin types. Melanosomes have a thermal relaxation time of 50-500 nanoseconds. [22, 23, 24] With pulse durations in the nanosecond range, historically these lasers provided the most destructive effects on melanosomes with the least damage to surrounding cellular structures. However 2018 studies by Negishi et al note that the picosecond laser can damage specific melanosome targets, while nanosecond lasers have more of a clustered target. [25] This additional specificity may be the science behind more recent studies reporting reduced PIPA with the use of picosecond lasers.
Other laser designs to enhance selective photothermolysis are based on wavelength and its relationship to frequency. Common wavelengths used to target melanin include 532 nm, 694 nm, 755 nm, and 1064 nm. For example, the potassium titanyl phosphate (KTP) laser, which has wavelength of 532 nm, is derived from a frequency-doubled Nd:YAG laser. [26]
Beginning with treatment of pigmented lesions, lentigines are the easiest [18] to treat and are the most responsive to lasers. When beginning treatment, initial laser settings should be based on the recommended factory settings. The initial response can help further guide the clinician for maximizing treatment. Understanding targeted tissue response in determination of a treatment endpoint is essential for optimum results; however, this end point varies depending on the laser used. While long-pulsed lasers typically show slight darkening of the pigmented lesion, the nanosecond and picosecond lasers demonstrate immediate whitening. [18, 25, 27] The immediate pigment whitening is attributed to steam bubbles in the epidermis that occur after treatment. Negishi et al suggest that given the lower fluence required for picosecond lasers, [25] a milder endpoint is also required. If the same endpoint is achieved, an increased risk of PIPA may follow. Simply following targeted endpoints does not alleviate the risk of PIPA, even in the absence of an adverse clinical event. Adverse tissue changes can manifest within 5 minutes of treatment and may be noted by light lateral pressure causing separation of the skin, termed the Nikolsky sign, which is representative of keratinocyte necrosis, [28] Although typically well tolerated, an unexpected elevation of pain during the procedure should alert the clinician for the potential of an adverse outcome.
A study by Imhof et al reported a split-face study comparing triple-combination cream (TC) and a nanosecond Q-switched 694-nm ruby laser. [22] The TC was based on the Kligman formula and contained 5% hydroquinone, 0.03% tretinoin, and 0.03% dexamethasone. The reported both treatments produced beneficial results; however, the Q-switched 694-nm ruby laser demonstrated faster, superior, and longer-lasting effects with only one or two treatments. The settings used for the nanosecond 694-nm laser included a 4-mm spot, 20 nanseconds, and 4 J/cm2 used in a single pass ensuring less than 10% overlap.
Bohnert et al report nanosecond dual-pulse 532-nm/1064-nm laser with statistically superior results from their split-face study of solar lentigines when compared with a nanosecond 1064-nm laser for a single treatment session in a small cohort of ten patients. [15] Additional limitations were noted in lighter skin types, Fitzpatrick I-III, and thus may not be statistically significant for all skin types or for a complete treatment course.
Café au lait macules (CALMs) and nevus spilus lesions are frequently encountered epidermal lesions. A nevus spilus is sometimes referred to as a speckled lentiginous nevus or a zosteriform lentiginous nevus and typically appears as a CALM that has scattered hyperpigmented macules within a lightly pigmented patch. They most commonly present on the trunk or extremities and can be seen following the lines of Blashko. [29] Similar to lentigines, multiple treatment approaches are available, including surgery, chemical peels, and intense pulsed light (IPL) in addition to laser-based modalities. [30] Common wavelengths used for CALMs include the 532 nm, 694 nm, and 755 nm, [18] using either nanosecond or picosecond lasers. Although picosecond lasers may require fewer treatments, both classes of lasers require multiple treatments. It is recommended a minimum of 8 weeks elapse between treatment sessions. [18]
As with treatment of any pigmented lesion, a conservative approach with a careful history is recommended to evaluate for neurofibromatosis or other similar genodermatosis. [18] Although rare, malignant potential has been reported in a case report describing a melanoma arising from a nevus spilus. [31]
Regarding laser treatments, Abrusci and Benzecry address the cosmetic burden that can be associated with a nevus spilus. [30] Their case report describes treatment of the cosmetically sensitive area of the neck. Four treatments were used at 3-month intervals. Twenty-four months after the fourth and final treatment, no recurrence was reported. The settings used were based on the size needed for spot treatment of a single pigmented macule (5 mm, 10 microseconds, 19 J/cm2 or 3 mm, 10 microseconds, 21 J/cm2). Two passes without cooling were conducted at each visit. On the second, third, and fourth visit, field treatment of the CALM was added (7 mm, 6 microseconds, 7.5 J/cm2) after spot treatment with the same settings previously noted for the initial visit. Additionally, no cooling was used for the field treatment and only a single pass was completed. Postoperative pain control was conducted though cold compresses.
Tang et al describe treatment of nevus spilus through a series of wavelength approaches. [29] They begin with a fractional ablative carbon dioxide laser, before following up with a nanosecond 1064-nm then 532-nm laser. Other studies have noted that the use of a fractional carbon dioxide laser may decrease blister formation and expedite healing in addition to enabling transepidermal elimination.
Seborrheic keratoses (SKs) are benign keratotic lesions that typically develop beginning in the fourth decade. Although SKs can appear on all areas of the body, sun exposure is still believed to be a critical part of development in a genetically susceptible patient. [31, 32] Dermatosis papulosa nigra (DPN), a variant of SK, is characterized by benign papules commonly located on the lateral face in persons of darker skin complexion, in particular Asian and African persons, [33] These lesions do not spontaneously resolve and often recur after treatment. Similar to previously discussed epidermal pigmented lesions, SKs and DPN respond readily to office procedures with liquid nitrogen, chemical peels, and electrosurgery, in addition to lasers-based treatment. The patients most commonly affected by DPN are the same population commonly affected by PIPA; therefore, careful consideration for the most safe and effective treatment is essential to treatment success. Laser use for the treatment of SKs continues to demonstrate efficacy while maintaining a higher safety profile. The treatment approach for SKs and DPN varies from the macular presentation to larger plaques that can develop; therefore, different settings should be implemented. Understanding appropriate tissue response is key when combining newer approaches to treatment.
Use of the pulsed-dye laser (PDL) is not commonly reported for treatment of SKs. Karadag et al report the use of a 585-nm PDL for the treatment of DPN, a variant of SKs. [33] They noted lesions on the face demonstrated over 75% improvement, while neck and trunk lesions had only 50-75% improvement over the same treatment protocol. Karadag et al report PDL settings with a 7-mm spot size, 8-9.5 J/cm2, and a pulse duration of 10 microseconds. Patients received two to six treatments at 4-week intervals.
Alegre-Sanchez used a picosecond 755-nm laser for SKs. [24] Depending on the morphology of the targeted lesions, settings and hand piece position varied. The larger papules required the hand piece to be held 3-5 cm above the lesion to ensure the energy was focused in the epidermis, while flat lesions maintained skin contact. They report 67% clearance at the completion of treatment using spot sizes of 2.5-3.5 mm with a fluence of 2-4 J/cm2.
Sayan et al used field treatment with the Er:YAG laser in 261 patients for the treatment of SKs with complexion types I-IV. [32] Using a 3- to 5-mm spot size, with 350-500 mJ/cm2 in a short-pulse mode, they predominately treated head and neck lesions. The Er:YAG laser at 2940 nm has 10-15 times greater water absorption when compared with traditional carbon dioxide lasers, enabling a more precise treatment with less collateral damage, [32] all of which further decreases required postoperative downtime. [2] Sayan et al report that 98% of patients had resolution of erythema by 2 weeks following treatment.
Treatment of epidermal lesions doe not come without risk. Multiple studies have reported higher complication rates from nanosecond lasers in the treatment of solar lentigines. [17, 34] In a multicenter study involving over 500 subjects, Kang et al reported over 20% of patients developing postinflammatory hyperpigmentation with treatment using nanosecond 532-nm frequency-doubled Nd:YAG laser. [34] They reported treatment of erythematous lentigines, velvety skin, or generalized dyschromia had a higher probability of developing PIPA. Wanner et al also reported that long-pulse lasers had less PIPA when compared with nanosecond lasers. [27]
Other approaches to reduce treatment-induced PIPA continue to be explored. Newer picosecond lasers are slowly proving their worth for treatment of pigmented lesions. Negishi et al report less than 5% postinflammatory hyperpigmentation in their smaller study of treatment for lentigines with a 532-nm picosecond laser. [25] In addition to the significantly reduced PIPA rate, 93% of treated patients reported greater than 75% clearance after a single treatment.
Melasma is a disorder of hyperpigmentation where the pigment can be located in the epidermis, dermis, or both. Studies involving the histology of melasma are contradictory regarding location of pigment, but it does appear that epidermal and dermal pigment contribute to the overall clinical picture. [35, 36, 37] Important to note is that these studies consistently showed that melanocytes in a patient appear more dendritic and with features that suggest higher activity. These features included increased melanosomes in general, increased stage III and IV melanosomes, more reaction product after the addition of dihydroxyphenylalanine (DOPA), more mitochondria, Golgi apparatus, rough endoplasmic reticulum, and ribosomes all when compared with normal perilesional biopsies from the same patients. [37]
The etiology of melasma is not well understood, but it does appear that UV radiation, hormonal therapies, pregnancy, cosmetics, certain medications, and genetic factors play a role. [23, 38] Additionally, thyroid disorders, [39] increased stem cell factor and c-kit expression, [40] increased nerve growth factor receptor, [41] and elevated vascular endothelial growth factor levels [42] add to the multifactorial presentation.
Melasma mostly commonly affects females, but it can also affect males. It preferentially affects those with Fitzpatrick skin types III and IV. The diagnosis is often straightforward, but pitfalls can occur. Melasma may mimic other common dermatological presentations, including drug-induced hyperpigmentation, lichen planus pigmentosus, ashy dermatosis, facial acanthosis nigricans, frictional melanosis, PIPA, solar lentigines, ephelides, and nevus of Ota. [43] There are many clinical patterns of melasma, but they typically present in a preferentially sun-exposed pattern.
The foundation of treatment and prevention of melasma begins with sun protection and avoidance of triggers. While the following discussion focuses on laser- and light-based modalities for treatment of melasma, there are many treatment options. Topical agents (eg, hydroquinone, retinoids, azelaic acid), chemical peels, oral tranexamic acid, and camouflage remain important parts of the therapeutic algorithm. Spierings suggests that laser treatment of melasma should be considered in patients who prefer more rapid resolution of pigmentary issues and in those in whom topical agents and/or chemical peels have failed. [23] Lasers are primarily considered second-line treatment, given the increased cost and reduced access when compared with other topical treatments. Historically, nanosecond lasers were considered the criterion standard. Many lasers are currently available, and newer research has demonstrated superior treatment options. As more research continues to be released on picosecond lasers, the standard may be changing. Regardless of the treatment approach, melasma continues to be a challenging therapeutic hurdle. Any treatment used, including laser- and light-based devices, can lead to worsening of the condition. [44] It is important to remember that prophylaxis treatment for PIPA with topical agents is important before starting laser-based treatment.
In an early study by Taylor and Anderson, [45] patients were treated with a QSRL at 694 nm with a pulse duration of 40 nanoseconds. Laser parameters were a 5-mm spot and 4.5-7.5 J/cm2; the number of passes or amount of overlap with pulse was not discussed. Unfortunately, most patients experienced no improvement, while two reported worsening of the condition. For this laser, the fluences of 4.5-7.5 J/cm2 would be considered standard fluence settings for epidermal pigment lesions. Further work with nanosecond lasers has shown some benefit with low-fluence protocols with multiple passes. In a study by Zhou et al, [46] 50 patients were treated with low-fluence nanosecond Nd:YAG laser at a wavelength of 1064 nm. Patients were treated at 1-week intervals for nine total treatments. Laser parameters used a 6-mm spot, 2.5-3.4 J/cm2 at 10 Hz. This was passed slowly over the areas of melasma for five passes or until slight erythema. The self-evaluated results showed 60% of patients reported greater than 75% clearance of the pigmentation and 10% rated a poor response (0-25% clearance). Unfortunately, 64% had relapse of the disease at the 3-month follow-up visit. Unlike the benefits of laser toning noted in lentigines, melasma patients have not responded as well. Although not reported in this study, laser toning has been associated with mottled hypopigmentation that may not self-resolve over time. [47]
In comparing a nanosecond Nd:YAG laser to a nanosecond Q-switched alexandrite laser (QSAL) at 755 nm, [48] Fabi et al showed benefit with both treatments in a split-face study. Six total treatment sessions were provided at 1-week intervals. Follow up was done at 2, 12, and 24 weeks after the treatment phase. Laser settings used in the treatment for the nanosecond Nd:YAG laser were 8-mm spot, 1-2 J/cm2 at 5 Hz, with one to eight passes until slight erythema developed. The nanosecond QSAL setup included a 6-mm spot defocused with an added 30 mm to the distance finger (simulating an 8-mm spot), and the fluence was set at 1.8 J/cm2 for an effective fluence of 1.1 J/cm2 at 5 Hz, one to two passes until mild erythema developed. Sunscreen was used after each treatment, and strict sun protection was advised. No significant differences were found between the treated sides. Patients with Fitzpatrick skin type II reported significantly greater improvement when compared with those with type IV skin. One patient developed bilateral PIPA and was removed from the study.
In a review in the Journal of the American Academy of Dermatology, nanosecond lasers were not a recommended form of treatment for patients with melasma. [44] However, in the authors’ opinion, low-fluence laser toning is a viable option after more conservative treatments have failed. Given the higher risk of PIPA with nanosecond lasers, patients must fully understand that adverse outcomes may occur and there is potential for irreversible cosmetic results. [49]
Choi et al report using a 750-picosecond dual-wavelength of 595 nm and 1064 nm with hydroquinone in a prospective split-face study compared with hydroquinone alone. [50] Their study noted that 76.92% of the 39 female patients with complexion type III-V reported greater than 51% improvement with the addition of the picosecond laser. [23, 50] Further, the recurrence rate remained low at the 12-week follow up. Variable settings were used to localize treatment areas. Initial pass was completed with the picosecond 1064-nm laser with ranges in spot size from 7-10 mm and fluence of 0.2-1.5 J/cm2 over two to four passes that were followed by a 595-nm laser, 0.1-0.55 J/cm2 fluence using a 5-mm spot with one to two passes until the presence of mild erythema.
Fractional ablative laser techniques create microscopic treatment zones within the epidermis. This approach creates microscopic epidermal debris [2] that can be removed through a restorative process such as transepidermal elimination. Nonablative fractional lasers (NAFLs) create microthermal zones (MTZs) of heating without ablating tissue. This leads to less downtime for the patient compared with ablative laser treatments, as no reepithelialization needs to occur. One study comparing 1550-nm NAFL to TC in a split-face model showed no statistical difference in physician global assessment scores. [51] On the NAFL-treated side, 31% of patients had postinflammatory hyperpigmentation. They suggested that 15-mJ per MTZ was not safe or effective in the treatment of melasma, which called into question the efficacy of NAFL for treatment of melasma.
The 1550-nm wavelength NAFL by Fraxel has been approved by the US Food and Drug Administration since 2005 for the treatment of patients with melasma. A pilot study using NAFL showed 60% of patients had 75-100% improvement after 4-6 treatment sessions separated by 1-2 two weeks. [52] Long-term follow up was not conducted, and recurrence was not a measured outcome. One of the 10 patients developed PIPA. Other studies using the same device have reproduced similar results. [53]
A newer wavelength platform, the thulium fiber 1927-nm NAFL, has higher avidity to water and is therefore absorbed at more superficial levels of the epidermis and dermis. In a pilot study, 14 patients were treated with 3-4 total sessions at 4-week intervals. [54] Settings of 10-20 mJ per MTZ were used, with an estimated surface area coverage of 20-45% with each treatment. At 1-month post treatment, Melasma Area and Severity Index (MASI) scores decreased by 51%. MASI scores remained decreased by 33% and 34% at 3- and 6-month follow-up, respectively. There were no cases of PIPA reported. A study using more high-density coverage with the same 1927-nm NAFL showed that one treatment session could produce effective results. [55] Settings used were 10-20 mJ per MTZ and at a total surface area coverage of 60-70%. Twenty patients were treated in this retrospective review. Sixty percent of patients had a 50% improvement or more at 4 weeks. PIPA, however, was noted in 10% of treated patients.
NAFL appears to be the best laser type for treating melasma when comparing the risk-benefit ratio. The authors believe this to be the case, given it rarely shows permanent scarring or pigment changes. Additionally, it is not a pigment-specific wavelength, as it targets water, and therefore can be used safely in all skin types. Some studies have followed patients for 6-12 months after NAFL and have shown some sustained benefit. Of the NAFL technology, the authors consider the 1927-nm thulium fiber NAFL to be the better wavelength for targeting the process more effectively. Picosecond lasers may show improved efficacy over nanosecond lasers, but further studies are needed before a determination can be rendered.
Ablative lasers are highly avid for the chromophore of water and therefore vaporize tissue. This technology can be subdivided into nonfractionated ablative lasers and ablative fractional lasers (AFLs). Postoperative downtime and procedural risks differ between these ablative laser therapies. Ablative laser treatment, otherwise known as full-field resurfacing, typically creates broad superficial ablation of the epidermis. Given the more aggressive approach, patients should expect a longer recovery period. AFLs create microablative channels in the skin and treat only a portion of the skin. This procedure is typically better tolerated and frequently has a decreased down time.
In a study by Manaloto and Alster, [56] an Er:YAG laser was used in nonfractionated ablative laser settings for full-field resurfacing in 10 patients with refractory melisma, all with skin types II-V. Improvement of MASI scores was noted after 1 week. However, postinflammatory hyperpigmentation invariably occurred leading to worsening of MASI scores by week three. Patients were then treated with glycolic acid peels, sunscreen use, and azelaic acid as tolerated. At six months, MASI scores had decreased from baseline. Given the near-universal adverse effect of postinflammatory hyperpigmentation, the authors suggested this approach should only be considered for refractory cases.
A novel variable square-pulsed (VSP) Er:YAG laser was used in a study by Wanitphakdeedecha et al. [57] Twenty patients were treated with two passes using a 7-mm spot, pulse duration of 300 microseconds, and fluence of 0.4 J/cm2. Less postinflammatory hyperpigmentation occurred in this study (17.6%), and the authors suggest this was a vast improvement when compared with previous studies with traditional pulsed Er:YAG systems.
AFL treatments have also been studied for the treatment of melasma. In a split-face study by Jalaly et al, [58] a low-fluence Nd:YAG treatment was compared to AFL treated with low-power carbon dioxide, at a wavelength of 10600 nm. Five total treatment sessions where completed at 3-week intervals. Both treatments created reduction in the modified MASI score. Although both laser approaches noted improvement, the reduction in modified MASI score was superior for the low-power AFL carbon dioxide laser.
The low-power AFL carbon dioxide laser has been studied more recently and showed reduced MASI scores after five treatment sessions. [59] Thirty patients were included in the study, and the entire diseased area was treated with AFL for five treatments 4-6 weeks apart. The laser parameters were power of 12 W, spacing of 800 mm (7.3% density), dwell time of 300 microseconds, stack 1, and frequency of 1 Hz. Interestingly, this study used topical tranexamic acid on one side of the face after treatment with AFL. MASI scores actually decreased more on the AFL-alone side. Low-power AFL may be a reasonable option for refractory melasma.
IPL is a light-based treatment that has been used for the treatment of melasma. It emits noncoherent wavelengths of 500-1200 nm and uses adjustable filters to narrow the treatment spectrum.
In a study by Wang et al, [60] 33 patients in whom at least 3 months of topical hydroquinone treatment failed were divided into two groups; 17 patients were treated with IPL for four total sessions separated by 4 weeks. The IPL group also received 4% hydroquinone and sunscreens. The control group received 4% hydroquinone and sunscreens only. A 570-nm cutoff filter was used in the first session, and 590- to 615-nm filters were used during subsequent sessions in an attempt to treat residual deeper pigment. Laser parameters were fluences of 26-33 J/cm2; double mode; and pulse durations of 3-4 and 4-5 microseconds, respectively, with a delay of 30-35 microseconds between pulses. Patients in the IPL treatment group were noted to have reduced Relative Melanin Index (RMI) scores that were significant compared with the control group. PIPA was reported in two patients from the IPL group.
Goldman et al studied IPL with and without TC in a 10-week split face study. [61] They demonstrated that IPL with TC has an important synergistic effect when compared with IPL alone. IPL and TC is a safe and effective treatment approach for moderate-to-severe melasma. Although IPL can be a useful tool in the treatment armamentarium for melasma, it is the authors’ opinion that other laser- and light-based approaches have demonstrated superior outcomes with less PIPA. Important to note is that settings, modes, and passes are widely variable from one device to another. There is less room for error in this type of device, and the practitioner should be well versed in the use of their device and its interactions with skin prone to hyperpigmentation.
Dermal melanocytosis has many clinical presentations, two of which are nevus of Ota and nevus of Ito. Nevus of Ota presents as blue-grey hyperpigmented macules and patches over the first and second trigeminal nerve distribution. This is typically found in a unilateral distribution; however, it may occur bilaterally—referred to as a Hori nevus. The differential diagnosis for a nevus of Ota includes drug-induced hyperpigmentation, lichen planus pigmentosus, erythema dyschromicum perstans, melasma, and PIPA. Nevus of Ito is a similar process that occurs on the upper back or shoulder. Both of these nevi occur most commonly in the Asian population and typically present early in childhood.
Laser treatment for the cosmetic improvement of nevus of Ota and Ito begins with nanosecond lasers, as picosecond lasers have only recently been implemented. Melanosomes are the chromophore target of which has a tissue relaxation time of 50-500 nanoseconds. Selective photothermolysis of the melanosome can be safely treated with nanosecond and picosecond lasers that use wavelengths targeting pigment.
Multiple studies note the nanosecond ruby laser (QSRL), at a wavelength of 694 nm, as a highly effective treatment for nevus of Ota and Ito. The long wavelength successfully targets the deep spindle cell–shaped dermal melanocytes. Histologically, these melanocytes appeared to be destroyed after treatment with this laser. Geronemus reported treatment in 15 patients with a nevus of Ota that required up to seven treatments with QSRL. [62] Complete clearing was noted in four patients, with significant lightening in the others.
In a large study by Watanabe and Takahashi, [63] more than 100 individuals were treated with the QSRL. They report that the degree of lightening was directly related to the number of treatments. Lightening of greater than 70% was seen in 33 of 35 individuals treated at least four times. Laser parameters used included a 4-mm square spot with fluence of 6 J/cm2, and pulse duration of 30 nanoseconds.
In another study of the QSRL, Kono et al evaluated the use of QSRL in the treatment of nevus of Ota in different age groups. [64] The laser parameters reported were a spot size of 4 mm, fluence of 5-7 J/cm2, and pulse duration of 30 nanoseconds. Forty-six children and 107 adults were treated at 3- to 4-month intervals. There were statistically significant differences between children and adults, including the number of treatment sessions for lesion clearance (3.5 and 5.9, respectively), as well as the rate of complications, including hyperpigmentation and hypopigmentation (4.8 and 22.4%, respectively). The authors concluded that earlier treatment reduces the number of adverse effects and the total treatment sessions needed.
Nanosecond alexandrite laser (QSAL), at a wavelength of 755 nm, has also been studied in the treatment for a nevus of Ota. Given the longer wavelength, this laser has a deeper penetration in exchange for less avidity to melanin when compared with the QSRL. In a large retrospective analysis, 806 patients with nevus of Ota were treated with QSAL. [65] Laser parameters included a 3-mm spot size, 3.8-4.8 J/cm2 fluence, and pulse duration of 60 nanoseconds. Results noted that 93.9 % of patients achieved complete clearance after an average of 5.2 sessions with the QSAL.
Kang et al found that depth of pigment was important in patient outcomes for their study, [66] which included histological evaluation. Fifty-five patients were treated with QSAL for three total sessions at 3-month intervals. They found that patients with pigment that was less than or equal to 1 mm in depth had better response to treatment. Eighty percent showed good or excellent clearing, with 55% reporting PIPA that universally resolved within 4 months. Only 49% showed excellent clearing. More treatment sessions may enable higher clearance rates.
The nanosecond Nd:YAG laser, with a wavelength of 1064 nm, has the longest of the wavelengths traditionally used to treat nevus of Ota and Ito. The longer wavelength allows for deeper penetration, likely treating the entire dermis. This wavelength is less avid for pigment when compared with the previously mentioned lasers. This is a disadvantage as the chromophore target is melanin. Although this is a safety feature for treatment in darker-skinned patients, it likely also leads to less uniform hypopigmentation.
In a study comparing the efficacy of QSAL and the nanosecond Nd:YAG laser, Chan and colleagues treated 40 patients in a split-site design, [67] treating half of the lesions with QSAL and the other half with nanosecond Nd:YAG laser. Laser parameters for the nanosecond Nd:YAG laser used a 2-mm spot, fluence of 7-9 J/cm2, and pulse duration of 6 nanoseconds. Settings for the QSAL also used a 2-mm spot; however, a fluence of 6-9 J/cm2 and pulse duration of 75 nanoseconds was implemented. Complication rates were similar. The authors noted the nanosecond Nd:YAG laser was slightly more effective in this study. A separate study also compared QSAL with nanosecond Nd:YAG laser for the treatment of bilateral nevus of Ota or Hori nevus, [68] using a split-face design. Laser parameters were similar to the previously mentioned study by Chan et al; however, a spot size of 3 mm was used with an appropriately reduced fluence for each treatment. Both lasers performed similarly in terms of efficacy and adverse effects.
Low-fluence nanosecond Nd:YAG laser has also been studied in an effort to reduce pain during treatment, [69] in a retrospective analysis of 31 patients. Laser parameters reported included a 7- to 8-mm spot, fluence of 1.9-5 J/cm2, and pulse duration of 5-10 nanoseconds While previous studies mentioned the above-used treatment with one pass to an endpoint of epidermal whitening, this study treated with two to four passes until mild erythema without petechiae developed. Treatments were also more frequent in this study, every 2-3 weeks versus every 12 weeks reported in the studies mentioned previously. Most patients had an excellent response between 6 and 32 total treatments. Interestingly, younger patients showed the need for less fluence in order to achieve similar results, suggesting that treating earlier with low fluence is a viable option.
Picosecond lasers represent a novel group of laser devices characterized by ultrashort, picosecond pulse duration. Compared with nanosecond lasers, picosecond lasers exert a greater photomechanical effect, presumably leading to an enhanced breakdown of small pigment particles, with less risk of PIPA.
QSAL was compared with picosecond alexandrite laser (PSAL), both at 755-nm wavelength. [70] This was a split-site study involving 56 patients with up to six treatment sessions at 12-week intervals. PSAL showed superior efficacy with fewer treatment sessions. Adverse effects of PIPA were also lower and less severe in the PSAL-treated sites.
Use of picosecond 532-nm and picosecond 1064-nm laser was reported by Wong three patients. [71] This laser also used a novel microlens array to create fractionated treatment. Although only a small study, two of three patients had an excellent response after six to nine treatments.
Overall, many lasers in the nanosecond and picosecond domains are affective for the treatment of nevus of Ota and Ito. The most commonly reported adverse effects were PIPA, but scarring can occur. Picosecond lasers may prove to be more efficacious, but at this time nanosecond lasers have the highest level of evidence.
Table 1. Common Lasers Available for Treatment of Pigmented Lesions (not an all-inclusive list; additional lasers may be available). (Open Table in a new window)
Lentigines, CALMs, benign melanocytic nevi, nevus of Ota, tattoos, drug-induced pigmentation, melasma (low fluence)
Lentigines, CALMs, benign melanocytic nevi, nevus of Ota, tattoos, drug-induced pigmentation, melasma (low fluence)
Lentigines, CALMs, benign melanocytic nevi, nevus of Ota, tattoos, drug-induced pigmentation, melasma (low fluence)
Lentigines, CALMs, benign melanocytic nevi, nevus of Ota, tattoos, drug-induced pigmentation, melasma (low fluence)
Lentigines, CALMs, benign melanocytic nevi, nevus of Ota, tattoos, drug-induced pigmentation, melasma (low fluence)
Lentigines, CALMs, benign melanocytic nevi, nevus of Ota, tattoos, drug-induced pigmentation, melasma (low fluence)
Lentigines, CALMs, benign melanocytic nevi, nevus of Ota, tattoos, drug-induced pigmentation, melasma (low fluence)
Table 2. Laser-Based Endpoint Targets. (Open Table in a new window)
Mild perilesional erythema and lesional graying
Table 3. Lentigines (reported settings based on manufacture recommendation and clinical experience). (Open Table in a new window)
Naval Medical Center San Diego
Naval Medical Center San Diego
Low fluence on special sites
Naval Medical Center San Diego
Table 4. CALMs and Nevus Spilus (reported settings based on manufacture recommendation and clinical experience). (Open Table in a new window)
All treatments had postoperative cooling
Naval Medical Center San Diego
Naval Medical Center San Diego
Active FX followed by 1064 nm then 532 nm
Table 5. SK and DPN (reported settings based on manufacture recommendation and clinical experience). (Open Table in a new window)
Table 6. Melasma (reported settings based on manufacture recommendation and clinical experience). (Open Table in a new window)
6-12 mJ/mB (or MTZ)
10-20 mJ/mB (or MTZ)
252-784 MTZs/cm2 (or 6-8 passes)
2.5-3.4 J/cm2 (5 passes)
1-2 J/cm2 (1-8 passes until slight erythema)
0.2-1.5 J/cm2 (2-4 passes)
0.1-0.55 J/cm2 (1-2 passes)
0.4 J/cm2 (160 mJ) (50% overlap)
14 -18 J/cm2 (double pulse, delay 10-30 microseconds)
Table 7. Nevus of Oto (reported settings based on manufacture recommendation and clinical experience). (Open Table in a new window)
5-7 J/cm2 (whitening)
3.8-4.8 J/cm2 (whitening)
6-9 J/cm2 (whitening)
Nanosecond Nd:YAG laser (1064 nm)
7-9 J/cm2 (whitening)
Nanosecond Nd:YAG laser (1064 nm)
7-8 J/cm2 (whitening)
Nanosecond Nd:YAG laser (1064 nm); low fluence
1.9-5 J/cm2 (2-4 passes to mild erythema)
Table 8. Adverse Endpoints. (Open Table in a new window)
Laser in a patient previously treated with gold
Incorrect alignment of laser or cooling
Residue on laser contact surface
Epidermal necrosis, incorrect settings, insufficient cooling
Anderson RR, Parrish JA. Selective photothermolysis: precise microsurgery by selective absorption of pulsed radiation. Science. 1983 Apr 29. 220 (4596):524-7. [QxMD MEDLINE Link].
Polder KD, Landau JM, Vergilis-Kalner IJ, Goldberg LH, Friedman PM, Bruce S. Laser eradication of pigmented lesions: a review. Dermatol Surg. 2011 May. 37 (5):572-95. [QxMD MEDLINE Link].
Alster TS. Complete elimination of large cafe-au-lait birthmarks by the 510-nm pulsed dye laser. Plast Reconstr Surg. 1995 Dec. 96(7):1660-4. [QxMD MEDLINE Link].
Alster TS, Williams CM. Café-au-lait macule in type V skin: successful treatment with a 510 nm pulsed dye laser. J Am Acad Dermatol. 1995 Dec. 33(6):1042-3. [QxMD MEDLINE Link].
Fitzpatrick RE, Goldman MP, Ruiz-Esparza J. Laser treatment of benign pigmented epidermal lesions using a 300 nsecond pulse and 510 nm wavelength. J Dermatol Surg Oncol. 1993 Apr. 19(4):341-7. [QxMD MEDLINE Link].
Grekin RC, Shelton RM, Geisse JK, Frieden I. 510-nm pigmented lesion dye laser. Its characteristics and clinical uses. J Dermatol Surg Oncol. 1993 Apr. 19(4):380-7. [QxMD MEDLINE Link].
Bogdan Allemann I, Goldberg DJ. Benign pigmented lesions. Curr Probl Dermatol. 2011 Aug 16. 42:81-96. [QxMD MEDLINE Link].
Polla LL, Margolis RJ, Dover JS, et al. Melanosomes are a primary target of Q-switched ruby laser irradiation in guinea pig skin. J Invest Dermatol. 1987 Sep. 89(3):281-6. [QxMD MEDLINE Link].
Dover JS, Margolis RJ, Polla LL, et al. Pigmented guinea pig skin irradiated with Q-switched ruby laser pulses. Morphologic and histologic findings. Arch Dermatol. 1989 Jan. 125(1):43-9. [QxMD MEDLINE Link].
Lee WJ, Han SS, Chang SE, et al. Q-Switched Nd:YAG Laser Therapy of Acquired Bilateral Nevus of Ota-like Macules. Ann Dermatol. 2009 Aug. 21(3):255-60. [QxMD MEDLINE Link]. [Full Text].
Jadotte YT, Schwartz RA. Melasma: insights and perspectives. Acta Dermatovenerol Croat. 2010 Jul. 18(2):124-9. [QxMD MEDLINE Link].
Chan JC, Shek SY, Kono T, Yeung CK, Chan HH. A retrospective analysis on the management of pigmented lesions using a picosecond 755-nm alexandrite laser in Asians. Lasers Surg Med. 2016 Jan. 48 (1):23-9. [QxMD MEDLINE Link].
Kasai K. Picosecond Laser Treatment for Tattoos and Benign Cutaneous Pigmented Lesions (Secondary publication). Laser Ther. 2017 Dec 31. 26 (4):274-281. [QxMD MEDLINE Link].
Adatto MA, Amir R, Bhawalkar J, Sierra R, Bankowski R, Rozen D, et al.New and Advanced Picosecond Lasers for Tattoo Removal.Curr Probl Dermatol.2017. 52:113–123.[QxMD MEDLINE Link].
Bohnert K, Dorizas A, Sadick N. A prospective, randomized, double-blinded, split-face pilot study comparing Q-switched 1064-nm Nd:YAG versus 532-nm Nd:YAG laser for the treatment of solar lentigines. J Cosmet Laser Ther. 2018 Nov - Dec. 20 (7-8):395-397. [QxMD MEDLINE Link].
Cohen L, Nanda S, Zaiac M. Desmoplastic Melanoma Arising after 1,064 nm q-Switched Nd:YAG Laser of a Suspected Solar Lentigo. Case Rep Dermatol Med. 2019. 2019:3907671. [QxMD MEDLINE Link].
Nam JH, Kim HS, Lee GY, Kim WS. Beneficial Effect of Low Fluence 1,064 nm Q-Switched Neodymium:Yttrium-Aluminum-Garnet Laser in the Treatment of Senile Lentigo. Ann Dermatol. 2017 Aug. 29 (4):427-432. [QxMD MEDLINE Link].
Hruza GJ, Avram MM. Lasers and Lights. 3rd ed. Saunders Elsevier; 2013.
Rogers T , Krakowski AC , Marino ML , Rossi A , Anderson RR , Marghoob AA .Navy and Lasers: Practical Considerations.Lasers Surg Med.2018 Jan.50(1):7-9[QxMD MEDLINE Link].
Hibler BP, Connolly KL, Lee EH, Rossi AM, Nehal KS. Lentigo maligna melanoma with a history of cosmetic treatment: Prevalence, surgical outcomes and considerations. Lasers Surg Med. 2017 Nov. 49 (9):819-826. [QxMD MEDLINE Link].
Chan MWM, Shek SY, Yeung CK, Chan HH. A Prospective Study in the Treatment of Lentigines in Asian Skin Using 532 nm Picosecond Nd:YAG Laser. Lasers Surg Med. 2019 Nov. 51 (9):767-773. [QxMD MEDLINE Link].
Imhof L, Dummer R, Dreier J, Kolm I, Barysch MJ. A Prospective Trial Comparing Q-Switched Ruby Laser and a Triple Combination Skin-Lightening Cream in the Treatment of Solar Lentigines. Dermatol Surg. 2016 Jul. 42 (7):853-7. [QxMD MEDLINE Link].
Spierings NMK. Melasma: A critical analysis of clinical trials investigating treatment modalities published in the past 10 years. J Cosmet Dermatol. 2019 Oct 11. [QxMD MEDLINE Link].
Alegre-Sanchez A, Jimenez-Gomez N, Moreno-Arrones OM, Fonda-Pascual P, Perez-García B, Jaen-Olasolo P, et al.Treatment of flat and elevated pigmented disorders with a 755-nm alexandrite picosecond laser: clinical and histological evaluation.Lasers With Sci.2018 Nov.33(8):1827–1831.[QxMD MEDLINE Link].
Negishi K, Akita H, Matsunaga Y. Prospective study of removing solar lentigines in Asians using a novel dual-wavelength and dual-pulse width picosecond laser. Lasers Surg Med. 2018 Oct. 50 (8):851-858. [QxMD MEDLINE Link].
Railan D, Parlette EC, Uebelhoer NS, Rohrer TE. Laser treatment of vascular lesions. Clin Dermatol. 2006 Jan-Feb. 24 (1):8-15. [QxMD MEDLINE Link].
Wanner M, Sakamoto FH, Avram MM, Chan HH, Alam M, Tannous Z, et al. Immediate skin responses to laser and light treatments: Therapeutic endpoints: How to obtain efficacy. J Am Acad Dermatol. 2016 May. 74 (5):821-33; quiz 834, 833. [QxMD MEDLINE Link].
Wanner M, Sakamoto FH, Avram MM, Anderson RR. Immediate skin responses to laser and light treatments: Warning endpoints: How to avoid side effects. J Am Acad Dermatol. 2016 May. 74 (5):807-19; quiz 819-20. [QxMD MEDLINE Link].
Tang M, Cheng Y, Yang C, Liu S, Sheng Y, Li Y, et al. Nevus spilus: treatment with fractional CO2 laser in combination with MedLite C6 laser: a preliminary study. Lasers Med Sci. 2017 Sep. 32 (7):1659-1662. [QxMD MEDLINE Link].
Abrusci V, Benzecry V. Medium-sized nevus spilus of the neck treated with pulsed dye laser. Dermatol Ther. 2017 Jul. 30 (4):[QxMD MEDLINE Link].
Bolognia J, Schaffer J, Cerroni L. Dermatology.4th ed.Elsevier;2017.
Sayan A, Sindel A, Ethunandan M, Ilankovan V. Management of seborrhoeic keratosis and actinic keratosis with an erbium:YAG laser-experience with 547 patients. Int J Oral Maxillofac Surg. 2019 Jul. 48 (7):902-907. [QxMD MEDLINE Link].
Karadag AS, Ozkanli Ş, Mansuroglu C, Ozlu E, Zemheri E. Effectiveness of the Pulse Dye Laser Treatment in a Caucasian Women With Dermatosis Papulosa Nigra.Indian J Dermatol.2015 May-Jun.60 ( 3 ):3[QxMD MEDLINE Link].
Kang HJ, Na JI, Lee JH, Roh MR, Ko JY, Chang SE. Postinflammatory hyperpigmentation associated with treatment of solar lentigines using a Q-Switched 532-nm Nd: YAG laser: a multicenter survey. J Dermatolog Treat. 2017 Aug. 28 (5):447-451. [QxMD MEDLINE Link].
Sanchez NP, Pathak MA, Sato S, Fitzpatrick TB, Sanchez JL, Mihm MC Jr. Melasma: a clinical, light microscopic, ultrastructural, and immunofluorescence study. J Am Acad Dermatol. 1981 Jun. 4 (6):698-710. [QxMD MEDLINE Link].
Grimes PE, Yamada N, Bhawan J. Light microscopic, immunohistochemical, and ultrastructural alterations in patients with melasma. Am J Dermatopathol. 2005 Apr. 27 (2):96-101. [QxMD MEDLINE Link].
Kang WH, Yoon KH, Lee ES, Kim J, Lee KB, Yim H, et al. Melasma: histopathological characteristics in 56 Korean patients. Br J Dermatol. 2002 Feb. 146 (2):228-37. [QxMD MEDLINE Link].
Grimes PE. Melasma. Etiologic and therapeutic considerations. Arch Dermatol. 1995 Dec. 131 (12):1453-7. [QxMD MEDLINE Link].
Lutfi RJ, Friedmanis M, Misiunas AL, Pafume O, Gonzalez EA, Villemur JA, et al.Association of melasma with thyroid autoimmunity and other thyroid abnormalities and their relationship to the origin of melasma.J Clin Endocrinol Metab.1985 Jul.61(1):28-3[QxMD MEDLINE Link].
Kang HY, Hwang JS, Lee JY, Ahn JH, Kim JY, Lee ES, et al. The dermal stem cell factor and c-kit are overexpressed in melasma. Br J Dermatol. 2006 Jun. 154 (6):1094-9. [QxMD MEDLINE Link].
Bak H, Lee HJ, Chang SE, Choi JH, Kim MN, Kim BJ. Increased expression of nerve growth factor receptor and neural endopeptidase in the lesional skin of melasma. Dermatol Surg. 2009 Aug. 35 (8):1244-50. [QxMD MEDLINE Link].
Kim EH, Kim YC, Lee ES, Kang HY. The vascular characteristics of melasma. J Dermatol Sci. 2007 May. 46 (2):111-6. [QxMD MEDLINE Link].
Sheth VM, Pandya AG. Melasma: a comprehensive update: part I. J Am Acad Dermatol. 2011 Oct. 65 (4):689-697. [QxMD MEDLINE Link].
Sheth VM, Pandya AG. Melasma: a comprehensive update: part II. J Am Acad Dermatol. 2011 Oct. 65 (4):699-714. [QxMD MEDLINE Link].
Taylor CR, Anderson RR. Ineffective treatment of refractory melasma and postinflammatory hyperpigmentation by Q-switched ruby laser. J Dermatol Surg Oncol. 1994 Sep. 20 (9):592-7. [QxMD MEDLINE Link].
Zhou X, Gold MH, Lu Z, Li Y. Efficacy and safety of Q-switched 1,064-nm neodymium-doped yttrium aluminum garnet laser treatment of melasma. Dermatol Surg. 2011 Jul. 37 (7):962-70. [QxMD MEDLINE Link].
Jang YH, Park JY, Park YJ, Kang HY. Changes in Melanin and Melanocytes in Mottled Hypopigmentation after Low-Fluence 1,064-nm Q-Switched Nd:YAG Laser Treatment for Melasma. Ann Dermatol. 2015 Jun. 27 (3):340-2. [QxMD MEDLINE Link].
Fabi SG, Friedmann DP, Niwa Massaki AB, Goldman MP. A randomized, split-face clinical trial of low-fluence Q-switched neodymium-doped yttrium aluminum garnet (1,064 nm) laser versus low-fluence Q-switched alexandrite laser (755 nm) for the treatment of facial melasma. Lasers Surg Med. 2014 Sep. 46 (7):531-7. [QxMD MEDLINE Link].
Shah SD, Aurangabadkar SJ. Laser Toning in Melasma. J Cutan Aesthet Surg. 2019 Apr-Jun. 12 (2):76-84. [QxMD MEDLINE Link].
Choi YJ, Nam JH, Kim JY, Min JH, Park KY, Ko EJ, et al. Efficacy and safety of a novel picosecond laser using combination of 1 064 and 595 nm on patients with melasma: A prospective, randomized, multicenter, split-face, 2% hydroquinone cream-controlled clinical trial. Lasers Surg Med. 2017 Dec. 49 (10):899-907. [QxMD MEDLINE Link].
Kroon MW, Wind BS, Beek JF, van der Veen JP, Nieuweboer-Krobotová L, Bos JD, et al. Nonablative 1550-nm fractional laser therapy versus triple topical therapy for the treatment of melasma: a randomized controlled pilot study. J Am Acad Dermatol. 2011 Mar. 64 (3):516-23. [QxMD MEDLINE Link].
Rokhsar CK, Fitzpatrick RE. The treatment of melasma with fractional photothermolysis: a pilot study. Dermatol Surg. 2005 Dec. 31 (12):1645-50. [QxMD MEDLINE Link].
Katz TM, Glaich AS, Goldberg LH, Firoz BF, Dai T, Friedman PM. Treatment of melasma using fractional photothermolysis: a report of eight cases with long-term follow-up. Dermatol Surg. 2010 Aug. 36 (8):1273-80. [QxMD MEDLINE Link].
Polder KD, Bruce S. Treatment of melasma using a novel 1,927-nm fractional thulium fiber laser: a pilot study. Dermatol Surg. 2012 Feb. 38 (2):199-206. [QxMD MEDLINE Link].
Niwa Massaki AB, Eimpunth S, Fabi SG, Guiha I, Groff W, Fitzpatrick R. Treatment of melasma with the 1,927-nm fractional thulium fiber laser: a retrospective analysis of 20 cases with long-term follow-up. Lasers Surg Med. 2013 Feb. 45 (2):95-101. [QxMD MEDLINE Link].
Manaloto RM, Alster T. Erbium:YAG laser resurfacing for refractory melasma. Dermatol Surg. 1999 Feb. 25 (2):121-3. [QxMD MEDLINE Link].
Wanitphakdeedecha R, Manuskiatti W, Siriphukpong S, Chen TM. Treatment of melasma using variable square pulse Er:YAG laser resurfacing. Dermatol Surg. 2009 Mar. 35 (3):475-81; discussion 481-2. [QxMD MEDLINE Link].
Jalaly NY, Valizadeh N, Barikbin B, Yousefi M. Low-power fractional CO₂ laser versus low-fluence Q-switch 1,064 nm Nd:YAG laser for treatment of melasma: a randomized, controlled, split-face study. Am J Clin Dermatol. 2014 Aug. 15 (4):357-63. [QxMD MEDLINE Link].
Tawfic SO, Abdel Halim DM, Albarbary A, Abdelhady M. Assessment of combined fractional CO2 and tranexamic acid in melasma treatment. Lasers Surg Med. 2019 Jan. 51 (1):27-33. [QxMD MEDLINE Link].
Wang CC, Hui CY, Sue YM, Wong WR, Hong HS. Intense pulsed light for the treatment of refractory melasma in Asian persons. Dermatol Surg. 2004 Sep. 30 (9):1196-200. [QxMD MEDLINE Link].
Goldman MP, Gold MH, Palm MD, Colón LE, Preston N, Johnson LA, et al. Sequential treatment with triple combination cream and intense pulsed light is more efficacious than sequential treatment with an inactive (control) cream and intense pulsed light in patients with moderate to severe melasma. Dermatol Surg. 2011 Feb. 37 (2):224-33. [QxMD MEDLINE Link].
Geronemus RG. Q-switched ruby laser therapy of nevus of Ota. Arch Dermatol. 1992 Dec. 128 (12):1618-22. [QxMD MEDLINE Link].
Watanabe S, Takahashi H. Treatment of nevus of Ota with the Q-switched ruby laser. N Engl J Med. 1994 Dec 29. 331(26):1745-50. [QxMD MEDLINE Link].
Kono T, Chan HH, Erçöçen AR, Kikuchi Y, Uezono S, Iwasaka S, et al. Use of Q-switched ruby laser in the treatment of nevus of ota in different age groups. Lasers Surg Med. 2003. 32 (5):391-5. [QxMD MEDLINE Link].
Liu J, Ma YP, Ma XG, Chen JZ, Sun Y, Xu HH, et al. A retrospective study of q-switched alexandrite laser in treating nevus of ota. Dermatol Surg. 2011 Oct. 37 (10):1480-5. [QxMD MEDLINE Link].
Kang W, Lee E, Choi GS. Treatment of Ota's nevus by Q-switched alexandrite laser : therapeutic outcome in relation to clinical and histopathological findings. Eur J Dermatol. 1999 Dec. 9 (8):639-43. [QxMD MEDLINE Link].
Chan HH, Ying SY, Ho WS, Kono T, King WW. An in vivo trial comparing the clinical efficacy and complications of Q-switched 755 nm alexandrite and Q-switched 1064 nm Nd:YAG lasers in the treatment of nevus of Ota. Dermatol Surg. 2000 Oct. 26 (10):919-22. [QxMD MEDLINE Link].
Wen X, Li Y, Jiang X. A randomized, split-face clinical trial of Q-switched alexandrite laser versus Q-switched Nd:YAG laser in the treatment of bilateral nevus of Ota. J Cosmet Laser Ther. 2016. 18 (1):12-5. [QxMD MEDLINE Link].
Seo HM, Choi CW, Kim WS. Beneficial effects of early treatment of nevus of Ota with low-fluence 1,064-nm Q-switched Nd:YAG laser. Dermatol Surg. 2015 Jan. 41 (1):142-8. [QxMD MEDLINE Link].
Ge Y, Yang Y, Guo L, Zhang M, Wu Q, Zeng R, et al. Comparison of a picosecond alexandrite laser versus a Q-switched alexandrite laser for the treatment of nevus of Ota: A randomized, split-lesion, controlled trial. J Am Acad Dermatol. 2019 Mar 16. [QxMD MEDLINE Link].
Wong THS. Picosecond Laser Treatment for Acquired Bilateral Nevus of Ota-like Macules. JAMA Dermatol. 2018 Oct 1. 154 (10):1226-1228. [QxMD MEDLINE Link].
Lentigines, CALMs, benign melanocytic nevi, nevus of Ota, tattoos, drug-induced pigmentation, melasma (low fluence)
Lentigines, CALMs, benign melanocytic nevi, nevus of Ota, tattoos, drug-induced pigmentation, melasma (low fluence)
Lentigines, CALMs, benign melanocytic nevi, nevus of Ota, tattoos, drug-induced pigmentation, melasma (low fluence)
Lentigines, CALMs, benign melanocytic nevi, nevus of Ota, tattoos, drug-induced pigmentation, melasma (low fluence)
Lentigines, CALMs, benign melanocytic nevi, nevus of Ota, tattoos, drug-induced pigmentation, melasma (low fluence)
Lentigines, CALMs, benign melanocytic nevi, nevus of Ota, tattoos, drug-induced pigmentation, melasma (low fluence)
Lentigines, CALMs, benign melanocytic nevi, nevus of Ota, tattoos, drug-induced pigmentation, melasma (low fluence)
Mild perilesional erythema and lesional graying
Naval Medical Center San Diego
Naval Medical Center San Diego
Low fluence on special sites
Naval Medical Center San Diego
All treatments had postoperative cooling
Naval Medical Center San Diego
Naval Medical Center San Diego
Active FX followed by 1064 nm then 532 nm
6-12 mJ/mB (or MTZ)
10-20 mJ/mB (or MTZ)
252-784 MTZs/cm2 (or 6-8 passes)
2.5-3.4 J/cm2 (5 passes)
1-2 J/cm2 (1-8 passes until slight erythema)
0.2-1.5 J/cm2 (2-4 passes)
0.1-0.55 J/cm2 (1-2 passes)
0.4 J/cm2 (160 mJ) (50% overlap)
14 -18 J/cm2 (double pulse, delay 10-30 microseconds)
5-7 J/cm2 (whitening)
3.8-4.8 J/cm2 (whitening)
6-9 J/cm2 (whitening)
Nanosecond Nd:YAG laser (1064 nm)
7-9 J/cm2 (whitening)
Nanosecond Nd:YAG laser (1064 nm)
7-8 J/cm2 (whitening)
Nanosecond Nd:YAG laser (1064 nm); low fluence
1.9-5 J/cm2 (2-4 passes to mild erythema)
Laser in a patient previously treated with gold
Incorrect alignment of laser or cooling
Residue on laser contact surface
Epidermal necrosis, incorrect settings, insufficient cooling
Curtis L Hardy, DO, FAAD CDR USN, Chair, Department of Dermatology, NMRTC San Diego Curtis L Hardy, DO, FAAD is a member of the following medical societies: American Academy of Dermatology, American Society of Dermatopathology, European Academy of Dermatology and Venereology Disclosure: Nothing to disclose.
James J Contestable, MD, MC, USN Director, Dermatology and Laser Clinic, Naval Medical Center Camp Lejeune; Assistant Professor of Clinical Dermatology, Uniformed Services University of the Health Sciences James J Contestable, MD, MC, USN is a member of the following medical societies: American Academy of Dermatology, American Society for Dermatologic Surgery, American Society for Laser Medicine and Surgery, American Society for MOHS Surgery, North Carolina Dermatology Association Disclosure: Nothing to disclose.
Kevin T Wright, MD, USN Staff Dermatologist, Naval Medical Center San Diego Disclosure: Nothing to disclose.
Michael J Wells, MD, FAAD Dermatologic/Mohs Surgeon, The Surgery Center at Plano Dermatology Michael J Wells, MD, FAAD is a member of the following medical societies: Alpha Omega Alpha, American Academy of Dermatology, American Medical Association, Texas Medical Association Disclosure: Nothing to disclose.
Dirk M Elston, MD Professor and Chairman, Department of Dermatology and Dermatologic Surgery, Medical University of South Carolina College of Medicine Dirk M Elston, MD is a member of the following medical societies: American Academy of Dermatology Disclosure: Nothing to disclose.
Tina S Alster, MD Clinical Professor, Department of Dermatology, Georgetown University School of Medicine; Director, Washington Institute of Dermatologic Laser Surgery Tina S Alster, MD is a member of the following medical societies: American Academy of Dermatology, American Medical Association, American Society for Dermatologic Surgery, American Society for Laser Medicine and Surgery, Medical Society of the District of Columbia Disclosure: Received ownership interest from Home Skinovations for other.
David J Goldberg, MD, JD Clinical Professor of Dermatology, Director of Laser Research, Icahn School of Medicine at Mount Sinai; Chief of Dermatologic Surgery, Chief of MOHS Surgery, Clinical Associate Professor of Dermatology, Rutgers New Jersey Medical School, Attending Physician, Department of Dermatology, Hackensack University Medical Center; Adjunct Professor of Law, Fordham University School of Law; Chief of Dermatologic Surgery, Department of Dermatology, Veterans Affairs Medical Center of East Orange, NJ David J Goldberg, MD, JD is a member of the following medical societies: American Academy of Dermatology, American College of Legal Medicine, American College of Mohs Surgery, American Society for Dermatologic Surgery, American Society for Laser Medicine and Surgery, European Academy of Dermatology and Venereology, International Society for Dermatologic Surgery, Skin Cancer Foundation Disclosure: Nothing to disclose.
Noah S Scheinfeld, JD, MD, FAAD † Assistant Clinical Professor, Department of Dermatology, Weil Cornell Medical College; Consulting Staff, Department of Dermatology, St Luke's Roosevelt Hospital Center, Beth Israel Medical Center, New York Eye and Ear Infirmary; Assistant Attending Dermatologist, New York Presbyterian Hospital; Assistant Attending Dermatologist, Lenox Hill Hospital, North Shore-LIJ Health System; Private Practice Noah S Scheinfeld, JD, MD, FAAD is a member of the following medical societies: American Academy of Dermatology Disclosure: Nothing to disclose.
Mary Farley, MD Dermatologic Surgeon/Mohs Surgeon, Anne Arundel Surgery Center
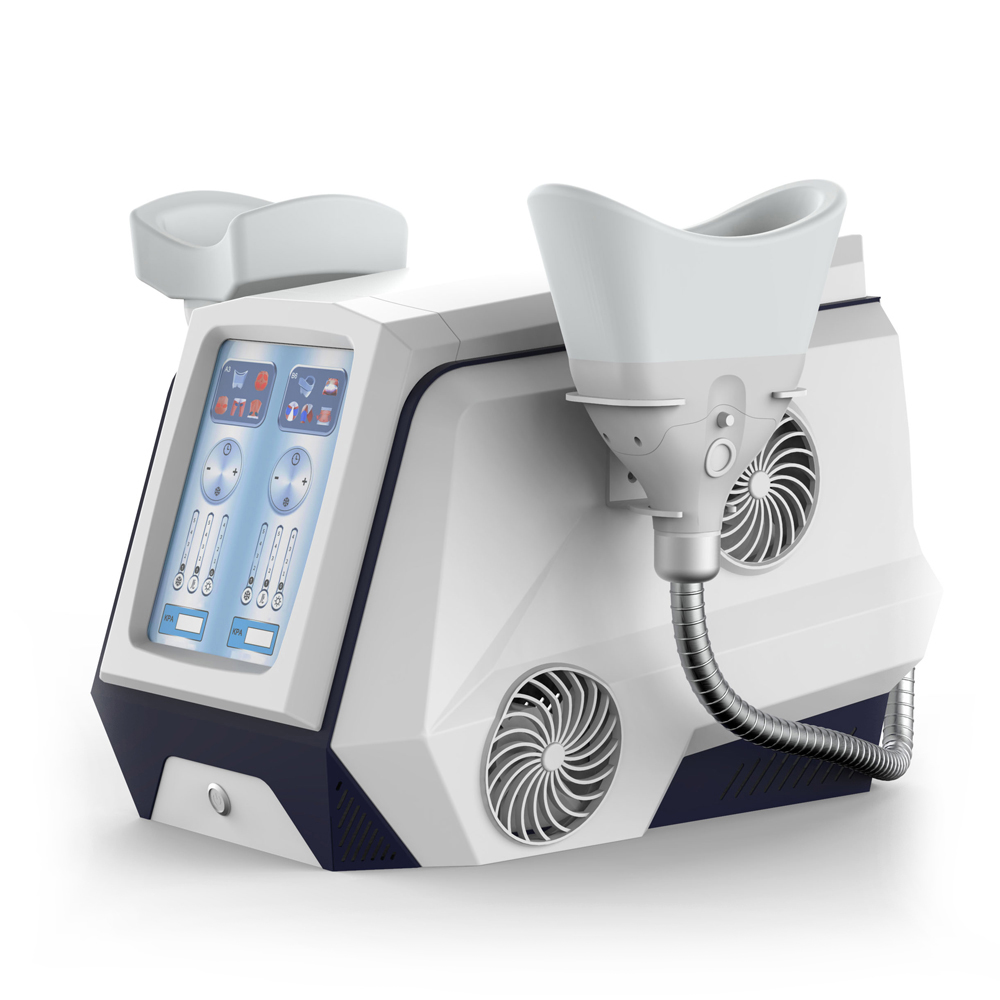
Diode Laser Machine Cost The views expressed in this article are those of the author(s) and do not necessarily reflect the official policy or position of the Department of the Navy, Department of Defense or the United States Government.The lead author is a military service member or employee of the Federal Government. This work was prepared as part of official duties. Title 17 U.S.C. 105 provides that ‘Copyright protection under this title is not available for any work of the United States Government.’ Title 17 U.S.C. 101 defines a United States Government work as a work prepared by a military service member or employee of the United States Government as part of that person’s official duties.