Thank you for visiting nature.com. You are using a browser version with limited support for CSS. To obtain the best experience, we recommend you use a more up to date browser (or turn off compatibility mode in Internet Explorer). In the meantime, to ensure continued support, we are displaying the site without styles and JavaScript.
It is over 60 years since Paul Cibis et al. reported the experimental use of liquid silicone in the surgical management of retinal detachment. Initial experiences were complicated by significant side-effects associated with the impurities in the non-medical grade commercial silicone oils deployed at the time. These were substantially reduced (but not eliminated) by the adoption of refined high-viscosity medical grade silicone oils. Two of the major complications associated with silicone tamponade are (i) the variability of focus due to its movement and higher refractive index, and (ii) progressive emulsification, particularly with low viscosity oils. This article reviews recent and ongoing research on the causes of emulsification of intra-ocular silicone oil to understand the causes better and thereby reduce this risk, especially for those eyes where permanent tamponade is the only current option for retaining vision. Casting Rubber

More than 60 years have passed since Paul Cibis and others experimentally used liquid silicone oil for the surgical treatment of retinal detachment.Initial experience was difficult to determine because the non-medical grade commercial silicone oils used at the time contained impurities that could produce serious side effects.By using refined, high-viscosity medical-grade silicone oil, side effects are greatly reduced (but not completely eliminated).The two main complications associated with silicone oil tamponade are: (i) changes in ocular focus due to movement and higher refractive index of silicone oil and (ii) progressive emulsification of silicone oil, especially low-viscosity silicone oil.This article reviews recent and ongoing research into the causes of intraocular silicone oil emulsification to better understand its causes and thereby reduce this risk, especially in patients for whom permanent tamponade is currently the only way to preserve vision.
Xihao Pan, Rui Li, … Hongwei Ouyang
Vision Loss Expert Group of the Global Burden of Disease Study & the GBD 2019 Blindness and Vision Impairment Collaborators
Tianyan Zhong, Hangjin Yi, … Xinyu Xue
It is over 60 years since Paul Cibis, Ed Okun and colleagues [1] reported the experimental use of liquid silicone in the surgical management of retinal detachment. Initial experiences were complicated by significant side-effects associated with the impurities in the non-medical grade commercial silicone oils deployed at the time and these were subsequently substantially reduced (but not eliminated) by the adoption of refined high-viscosity medical grade silicone. Two of the major complications associated with silicone tamponade are the variability of focus due to its movement and higher refractive index and progressive emulsification, particularly with low viscosity oils. For these reasons, removal of silicone oil tamponade is generally advisable as soon as the retina has been successfully stabilised. In a small minority of patients (particularly those with complex recurrent detachment), permanent silicone tamponade sometimes offers the only chance of salvage and stabilisation of vision and in such instances deployment of high purity, high viscosity oil is recommended [2] but progressive emulsification persists in some patients. Furthermore, the recent trend towards small gauge instrumentation has led some centres to revert back to use of low viscosity oils for ease of delivery and removal through narrow gauge cannulas but offset by risk of earlier and more extensive emulsification. This article reviews recent and ongoing research on the causes of emulsification of intra-ocular silicone oil to better understand and reduce this risk, especially for those eyes where permanent tamponade is the only current option for retaining vision.
Intraocular tamponades (IOTs) are used in vitreoretinal surgery to tamponade and maintain retinal break closure until the retinopexy has matured to full strength. Gaseous intraocular tamponades undergo spontaneous resolution by absorption into the blood stream (their volume being taken up by aqueous phase) whereas silicone tamponades require a second surgical intervention for their removal from the eye. Gaseous intraocular tamponades in common use include air, sulphur hexafluoride (SF6), perfluoroethane (C2F6) and perfluorooctane (C3F8). Liquid tamponades (often silicone oil (SO): polydimethyl siloxane, PDMS, with chain lengths of order 37–65 kDa [3]; corresponding to 450–850 monomers) are effectively immiscible with water. The eye continues to generate the aqueous phase from the ciliary body epithelium and aqueous leaves the eye principally via anterior chamber drainage system but also posteriorly (uveo-scleral outflow). In the vitreous cavity the aqueous phase forms a curved interface between either the gas or silicone tamponade. The shape of the static interface is determined by the level of fill, the contact angle between the two phases, θ, and the retinal wall, the local surface topology (e.g. smooth – lens; undulating – retina; shaped – scleral buckle) and the liquid properties: notably the difference in density of the two fluids, ∆ρ, and the interfacial tension, γ. Figure 1 illustrates the impact of these properties on interface shape for tamponade fluids in regular use and a 90% level of fill. The characteristic length scale is the capillary length, \({l}_{{{{{{\rm{c}}}}}}}\equiv \sqrt{\gamma /g\Delta \rho }\) , where g is the gravitational acceleration, and the position of the tamponade is determined by its density relative to aqueous.
a SO, density 0.965 g cm−3, contact angle, = 20°, interfacial tension (IFT) 35.5 mN m−1, 10.2 mm; b SO in (a) with = 15°; c heavy SO, density 1.06 g cm−3, = 20°, IFT 40.8 mN m−1, 8.2 mm; d gaseous tamponade (SF6), density 0.0065 g cm−3, = 20°, IFT 72.8 mN m−1, 2.7 mm. Aqueous phase in blue. Physical properties taken from the literature, values and methodology reported in Supplementary Material.
Developments in IOT technology over the last decade include the development of new tamponade materials, particularly silicone oil-based mixtures which are denser than water (e.g. Densiron 68, [4]) and hydrogel-based intravitreal tamponades [5, 6] with the objective of effectively tamponading inferior retinal breaks (on the lower part of the eyeball) as an alternative to the combination of PDMS IOTs and a scleral buckle. More recently, foldable capsular vitreous bodies (FCVBs) [7, 8] have been described to function as an intraocular balloon, inserted after pars planar vitrectomy then filled with liquid, providing a sterile and immobile tamponade.
Other studies include investigations of tamponade biocompatibility [9] and interactions between tamponade liquids and the aqueous phase.
The observation of emulsification continues to attract attention, as the origins of this phenomenon are still not well understood. In SOE, small droplets of SO are generated in the aqueous phase, impairing vision and potentially leading to complications. Table 1 summarises retrospective studies of tamponade complications over the past decade. Most of the studies featured small sample sizes or were drawn from one hospital or patients of one surgeon. The probability of emulsification in large studies is approximately 1 in 20 (5%). The question posed at the COS 2023 conference ‘Engineering and the Eye’ was ‘Why is the rate so low?’ and this paper focuses on the advances in understanding of the origins of SOE.
Table 1 shows that the average time for SOE to be observed in large studies increases with time for more viscous oils. The difference is not statistically significant, however. Chan et al. [10] reported the converse for their small (N = 9) study, which focused on the of use of a Coulter Counter (electrozone method) to determine the presence and size of SO droplets, while Yu et al. [11] reported noticeably shorter times (of order 6 months) for detection of SOE. These durations were similar to those reported by ultrasound biomicroscopy [12], SD-OCT [13] and slit lamp, gonioscopy and indirect ophthalmoscopy [14]. The method of inspection affects the detection efficiency noticeably. Emulsification is normally identified and quantified by optical observation, which sets a lower limit on the droplet size that can be detected, of approximately 30 μm. Chan et al. [10] and Yu et al. [11] measured droplet sizes in extracted fluids using techniques including laser light scattering and electrozone sensing, and reported sizes between 1 and 12 μm (with many 1–2 μm droplets), suggesting that SOE could be more prevalent than reported as smaller droplets are not detected by visual inspection. Romano et al. [15] recently proposed a grading system for SOE to allow systematic comparison between different studies.
Recent years have seen an increasing trend towards small gauge vitrectomy instrumentation, needing greater force for transfer of viscous oils and resulting in slower delivery: the scope for avoiding SOE by using highly viscous oils is limited. This has led to some centres reverting back to the use of low viscosity silicone oils and a resurgence of the attendant problems of rapid emulsification. Understanding the reasons for emulsification, thereby allowing mitigation to be planned for likely cases, has driven much of the research in this area.
The mechanism(s) causing SOE have yet to be established. Silicone oil and other immiscible liquids will form droplets in the aqueous phase when the interface between the two fluids becomes unstable. Adsorption of proteins and other surface active species at the interface will reduce the interfacial tension (IFT) – a measure of the work required to deform the interface - and promote interface breakup. Figure 1 shows how reducing the IFT changes the shape of the stable interface.
The flow conditions in the eye which promote breakup are not well understood. Motion of the two fluids in the posterior segment (see Fig. 2) is driven by wall drag, where the saccadic motion of the eyeball accelerates the fluid in contact with the retina. The local change in velocity is determined by the dynamic viscosity of the fluid (the ratio of viscosity to density, ν) which differs by a factor of ~103 between the aqueous and IOT phases. This leads to interfacial deformation. Detailed modelling of these flows, including computational fluid dynamics (CFD) simulations of the eyeball geometry, have been reported [16].
a Vertical section through midplane; b horizontal section through plane AA.
Figure 2 shows the three regions where different interfacial breakup mechanisms could be expected to occur:
Bulk deformation. Here, saccadic motion generates an unstable interface (Fig. 3) which is thought to shed droplets and is often cited as the cause of SOE [17]. Wang et al. [18, 19] have shown experimentally that the interface deformation induced by saccadic motion under simulated physiological conditions (saccade speeds, presence of surfactants, surface features such as the lens and scleral buckle) is not strong enough to cause emulsification of standard surgical SOs. This finding is consistent with the relatively low occurrence of SOE: if this mechanism was responsible, the expected probability would be closer to 100%.
Thin film rupture. Here, the aqueous layer at the side of the eye is subject to high shear rates as it transfers momentum to the SO sac. Chen et al. [20] postulated that the stability of the interface, when reduced by adsorption of surface active species, would lead to emulsification. The criterion for the stability of a thin layer of a less viscous liquid between a wall subject to periodic motion and a more viscous fluid was investigated by Isakova et al. [21], but these workers did not consider the ratio of viscosities (of order 103) which arise with IOTs associated with SOE. Moreover, these films are expected to present with all liquid tamponades.
Contact line disturbances. Wang et al. [19] observed droplet formation occasionally, in the region near the moving contact line. They hypothesised that emulsification could arise from motion of the two-liquid contact line across heterogeneous surface features (see Fig. 4). The motion of a two fluid contact line across surface heterogeneities, either arising from surface morphology or wettability, is the subject of active research in the fluid mechanics and microfluidics communities [22]. Wang [23] postulated that variations in the retinal surface, either arising from local morphology (e.g. scarring) or chemistry (adsorption of contaminants from the tamponade; local physiology) which rendered the surface more strongly wetting towards SO, could result in pinning of the contact line at these features, resulting in isolation of individual droplets, and their subsequent detachment when exposed to the high shear rates generated in the aqueous film.
a Stationary and b strongly agitated, saccadic motion amplitude 36°, 600° s−1. Experimental conditions: 1 Pa s silicone oil (L1) and 1 wt.% TX-100 saline solution (L2), volume ratio 91:9 SO: aqueous. [23], reproduced with permission.
The contact line moves across the site in (i–iii). As it retreats (iv-vi) it forms a filament which in this case (vii) ruptures to form a droplet (D) and an isolated SO drop (I). Alternately, a large isolated drop could be formed which subsequently gives daughter droplets as a result of high shear rates in the aqueous film.
Figure 5 shows some results of a synthetic experimental case, where motion of the contact line between the SO and aqueous phases across a solid surface featuring small hydrophobic patches results in droplet formation at the patches. Droplet formation was found to be determined by contact line speed, patch chemistry, patch shape and orientation (see Supplementary Information): these factors offer an explanation for the variation in observation of SOE between different individuals. It also offers insight into why the extensional viscosity of the SO can play a role in emulsification, as reported by Williams et al. [24]: the stretching of a droplet in a shear flow until it forms a neck which then breaks (Fig. 4(vi)) is governed by the shear stress imposed by the aqueous liquid and the extensional viscosity of the tamponade fluid. Wang did not observe droplet detachment often in these tests: this is consistent with the findings in studies of the detachment of silicone oil droplets from partially wetting (glass) surfaces by a shear flow of water and reported threshold relative velocities for droplet detachment [25], which Wang’s studies did not reach (see Supplementary Information). Testing this hypothesis for the parameter range of interest is the subject of ongoing research in our group.
a Experimental configuration: more information is given in the Supplementary Information. A glass microscope slide is initially plunged downwards at speed U so that the contact line is below its equilibrium location shown in (b). The contact line then climbs back up, the aqueous displacing the SO, recorded in the photographs in (c). The times in (c) indicate the time elapsed since the slide was moved downwards. The horizontal dashed yellow line indicates the location of a line of 50 μm diameter silane (trichloro-1H,1H,2H,2H-perfluoro-octyl-silane) dots on the slide. At t = 3.7 s the contact line has reached the line of dots as it moves upwards. It is pinned at the line (t = 6.7 s) and then detaches from the final dot, forming a rivulet (t = 11.7 s) which can break to give mobile drops (marked S) and stranded drops (e.g., P) which could then be sheared off subsequently (see Fig. 2). The shear rates required to cause detachment are discussed in Supplementary Material.
Mechanisms such as (3), which feature interactions at the small scale, mean that they can be probed using simplified geometries and the full complexity of the flow patterns generated in the eye do not need to be replicated, whereas studies of bulk mechanisms (e.g. (1)) do need to replicate local shear rates. The flow patterns generated by the saccadic motion in the eye are complex [26, 27] and some researchers have employed simplified 1-D and 2-D geometries to study SOE. Translating the results from these model systems to clinical practice is challenging as the flow patterns often do not map directly to the quasi-spherical geometry of the eye. Chan et al. [28] employed a horizontal cylinder (diameter 20 mm, length 20 mm) to study the effect of factors such as a scleral buckle on emulsification, but did not consider how the circulation flow and boundary layers generated by the moving walls are very different from those in the eye.
The 1-D ‘eye-on-a-chip’ presented by Chan et al. [29], featuring a cavity with height 1 mm and diameter 25 mm, allowed retinal ganglion (RGC-5) cells to be grown on the cylindrical walls, but the narrow aspect ratio meant that the liquids were subject to solid body rotation driven by the base and roof of the cavity rather than wall drag (Fig. 6) [30]. The shear rates generated in the wall film (region 2, Fig. 2) then differ significantly from those which arise in the eyeball.
The saccadic motion is modelled as a square wave shown in (a), with rotational velocity cycling between \(\pm {\omega }_{\max }\) , duration D and latency time 3D so that T = 4D. b shows the geometries: the equatorial plane is shaded. The velocity profiles in (c) show the scaled rotational velocity (local value divided by the maximum velocity reached at the wall) at different radial positions. Black loci show the velocity profiles for 0.5 Pa s SO (Womersley number, Wo = 0.54) and aqueous, Wo = 25.3, at time points indicated in (a), for a saccade displacement of 5.4° and \({\omega }_{\max }\) = 200 °s−1. Low Wo indicates that viscous effects determine the time response. The red locus shows the velocity profile for solid body rotation, which Mulcahy et al. [30] observed in the ‘eye-on-a-chip’ geometry of [29]. The two geometries exhibit significantly difference histories. Based on [21], reproduced with permission.
The advantages of lower viscosity silicone oils, in terms of ease of use (particularly with small gauge vitrectomy instrumentation) need to be offset by their reported greater speed and degree of emulsification when compared with higher viscosity oils. In addition, clinical observation suggests that aside from the viscosity of silicone oil, the speed and degree of emulsification varies widely between patients. Recent research challenges the historic concept of emulsification being due simply to bulk deformation of the silicone reservoir and indicates local disturbance of the contact line across differing biological or physical areas (natural, pathological or surgically induced e.g. scleral buckling) as a potential contributory factor. Further studies of the interaction of such biological and physical influences on the initiation of emulsification at the silicone oil/saline/tissue contact line should inform future strategies to reduce this complication. This is the subject of ongoing research.
Cibis PA, Becker B, Okun E, Canaan S. The use of liquid silicone in retinal detachment surgery. Arch Ophthalmol. 1962;68:590–59.
Article CAS PubMed Google Scholar
Hussain RN, Myneni J, Stappler T, Wong D. Polydimethyl siloxane as an internal tamponade for vitreoretinal surgery. Ophthalmologica. 2017;238:68–73.
Article CAS PubMed Google Scholar
Chan YK, Ng CO, Knox PC, Garvey MJ, Williams RL, Wong D. Emulsification of silicone oil and eye movements. Invest Ophthalmol Vis Sci. 2011;52:9721–7.
Article CAS PubMed Google Scholar
Davidson M, Dowlut S, Zhang J, Naderi K, Sandinha T, Wood MK, et al. Heavy silicone oil tamponade: a multicentre experience. BMJ Open Ophthalmol. 2022;7:e001018 https://doi.org/10.1136/bmjophth-2022-001018.
Article PubMed PubMed Central Google Scholar
Annaka M, Mortensen K, Vigild ME, Matsuura T, Tsuji S, Ueda T, et al. Design of an injectable in situ gelation biomaterials for vitreous substitute. Biomacromol. 2011;12:4011–21.
Baino F. Towards an ideal biomaterial for vitreous replacement: historical overview and future trends. Acta Biomater. 2011;7:921–35.
Article CAS PubMed Google Scholar
Lin X, Wang Z, Jiang Z, Long C, Liu Y, Wang P, et al. Preliminary efficacy and safety of a silicone oil-filled foldable capsular vitreous body in the treatment of severe retinal detachment. Retina. 2012;32:729–41.
Zhang X, Tian X, Zhang B, Guo L, Li X, Jia Y. Study on the effectiveness and safety of Foldable Capsular Vitreous Body implantation. BMC Ophthalmol. 2019;19:260 https://doi.org/10.1186/s12886-019-1268-x.
Article CAS PubMed PubMed Central Google Scholar
Romano MR, Ferrara M, Nepita I, D'Amato Tothova J, Giacometti Schieroni A, Reami D, et al.Biocompatibility of intraocular liquid tamponade agents: an update.Eye (London).2021;35:2699–713.
Article PubMed PubMed Central Google Scholar
Chan YK, Cheung N, Chan WS, Wong D. Quantifying silicone oil emulsification in patients: are we only seeing the tip of the iceberg? Graefes Arch Clin Exp Ophthalmol. 2015;253:1671–5.
Article CAS PubMed Google Scholar
Yu J, Zong Y, Jiang C, Zhu H, Deng G, Xu G. Silicone oil emulsification after vitrectomy for rhegmatogenous retinal detachment. J Ophthalmol. 2020: 6940625. https://doi.org/10.1155/2020/6940625.
Zhao H, Yu J, Zong Y, Jiang C, Zhu H, Xu G. Characteristics of silicone oil emulsification after vitrectomy for rhegmatogenous retinal detachment: An ultrasound biomicroscopy study. Front Med (Lausanne). 2022;8:794786.
Odrobina D, Laudańska-Olszewska I. Analysis of the time and location of the silicone oil emulsification by spectral-domain optical coherence tomography after silicone oil tamponade. Biomed Res Int. 2014;2014:372045. https://doi.org/10.1155/2014/372045.
Article CAS PubMed PubMed Central Google Scholar
Ratanapakorn T, Thongmee W, Meethongkam K, Sinawat S, Sanguansak T, Bhoomibunchoo C, et al. Emulsification of different viscosity silicone oil in complicated retinal detachment surgery: a randomized double-blinded clinical trial. Clin Ophthalmol. 2020;14:359–67.
Article PubMed PubMed Central Google Scholar
Romano MR, Ferrara M, Coco-Martin RM, Rickmann A, Spitzer MS, Steel DHW, et al. Intraocular emulsion of silicone oil (ITEMS) grading system: an evidence-based expert-led consensus. Retina. 2023;43:1370–6.
Rossi T, Querzoli G, Badas MG, Angius F, Telani S, Ripandelli G. Computational fluid dynamics of intraocular silicone oil tamponade. Transl Vis Sci Technol. 2021;10:22.
Article PubMed PubMed Central Google Scholar
Caramoy A, Schröder S, Fauser S, Kirchhof B. In vitro emulsification assessment of new silicone oils. Br J Ophthalmol. 2010;94:509–12.
Wang R, Snead M, Alexander P, Wilson DI. Assessing bulk emulsification at the silicone oil - saline solution interface in a 3D model of the eye. Acta Ophthalmol. 2021;99:e209–14.
Article CAS PubMed Google Scholar
Wang R, Tsai JH, Snead MP, Alexander P, Wilson DI. Stability of the interface between two immiscible liquids in a model eye subject to saccadic motion. J Biomech Eng. 2022;144:051004.
Chen Y, Kearns VR, Zhou L, Sandinha T, Lam WC, Steel DH, et al. Silicone oil in vitreoretinal surgery: indications, complications, new developments and alternative long-term tamponade agents. Acta Ophthalmol. 2021;99:240–50.
Article CAS PubMed Google Scholar
Isakova K, Pralits JO, Repetto R, Romano MR. A model for the linear stability of the interface between aqueous humor and vitreous substitutes after vitreoretinal surgery. Phys Fluids. 2014;26:124101–13.
Snoeijer JH, Andreotti B. Moving contact lines: scales, regimes, and dynamical transitions. Ann Rev Fluid Mech. 2013;45:269–92.
Wang R, Investigating silicone oil emulsification in eye chamber models. PhD Dissertation, University of Cambridge. 2022; https://doi.org/10.17863/CAM.82049.
Williams RL, Day M, Garvey MJ, English R, Wong D. Increasing the extensional viscosity of silicone oil reduces the tendency for emulsification. Retina. 2010;30:300–4.
Seeveratnam GK, Ding H, Michel O, Heng JYY, Matar OM. Laminar flow deformation of a droplet adhering to a wall in a channel. Chem Eng Sci 2010;65:4523–34.
Stocchino A, Repetto R, Siggers JH. Mixing processes in the vitreous chamber induced by eye rotations. Phys Med Biol. 2010;55:453–67.
Bonfiglio A, Lagazzo A, Repetto R, Stocchino A. An experimental model of vitreous motion induced by eye rotations. Eye Vis (Lond). 2015;2:10.
Chan YK, Cheung N, Wong D. Factors influencing the shear rate acting on silicone oil to cause silicone oil emulsification. Invest Ophthalmol Vis Sci. 2014;55:7451–6.
Chan YK, Sy KH, Wong CY, Man PK, Wong D, Shum HC. In vitro modeling of emulsification of silicone oil as intraocular tamponade using microengineered Eye-on-a-Chip. Invest Ophthalmol Vis Sci. 2015;56:3314–9.
Article CAS PubMed Google Scholar
Mulcahy MJ, Tremellen E, Alexander P, Snead MP, Wang R, et al. A critique of the ‘Eye on a Chip’ for assessing emulsification caused by silicone oil tamponades. Chem Eng Res Des. 2023;193:677–84.
Toklu Y, Cakmak HB, Ergun SB, Yorgun MA, Simsek S. Time course of silicone oil emulsification.Retina.2012;32:2039–44.
Article CAS PubMed Google Scholar
De Simone JD, Williamson JE, Mahmoudzadeh R, Wakabayashi T, Anderson HE, DeYoung C, et al. Incidence and complications of silicone oil emulsification after vitrectomy for complex retina diseases: comparison of 1000- versus 5000-centistoke silicone oil. Invest Ophthalmol Vis Sci. 2023;64:4548.
No external funding was received. A PhD studentship for author te Water Naude from the WD Armstrong Fund at the University of Cambridge is gratefully acknowledged.
These authors contributed equally: D. Ian Wilson, Andrew D. te Water Naude, Martin P. Snead.
Department of Chemical Engineering and Biotechnology, University of Cambridge, Philippa Fawcett Drive, Cambridge, CB3 0AS, UK
D. Ian Wilson & Andrew D. te Water Naude
Vitreoretinal Service, University of Cambridge, Addenbrookes Hospital, Hills Road, Cambridge, CB2 2QQ, UK
You can also search for this author in PubMed Google Scholar
You can also search for this author in PubMed Google Scholar
You can also search for this author in PubMed Google Scholar
ADT and DIW generated the initial manuscript and figures: all authors contributed to the review and finalisation of the manuscript.
Correspondence to D. Ian Wilson.
The authors declare no competing interests.
Publisher’s note Springer Nature remains neutral with regard to jurisdictional claims in published maps and institutional affiliations.
Open Access This article is licensed under a Creative Commons Attribution 4.0 International License, which permits use, sharing, adaptation, distribution and reproduction in any medium or format, as long as you give appropriate credit to the original author(s) and the source, provide a link to the Creative Commons licence, and indicate if changes were made. The images or other third party material in this article are included in the article’s Creative Commons licence, unless indicated otherwise in a credit line to the material. If material is not included in the article’s Creative Commons licence and your intended use is not permitted by statutory regulation or exceeds the permitted use, you will need to obtain permission directly from the copyright holder. To view a copy of this licence, visit http://creativecommons.org/licenses/by/4.0/.
Wilson, D.I., te Water Naude, A.D. & Snead, M.P. Refinements in the use of silicone oil as an intraocular tamponade. Eye (2024). https://doi.org/10.1038/s41433-024-03027-y
DOI: https://doi.org/10.1038/s41433-024-03027-y
Anyone you share the following link with will be able to read this content:
Sorry, a shareable link is not currently available for this article.
Provided by the Springer Nature SharedIt content-sharing initiative
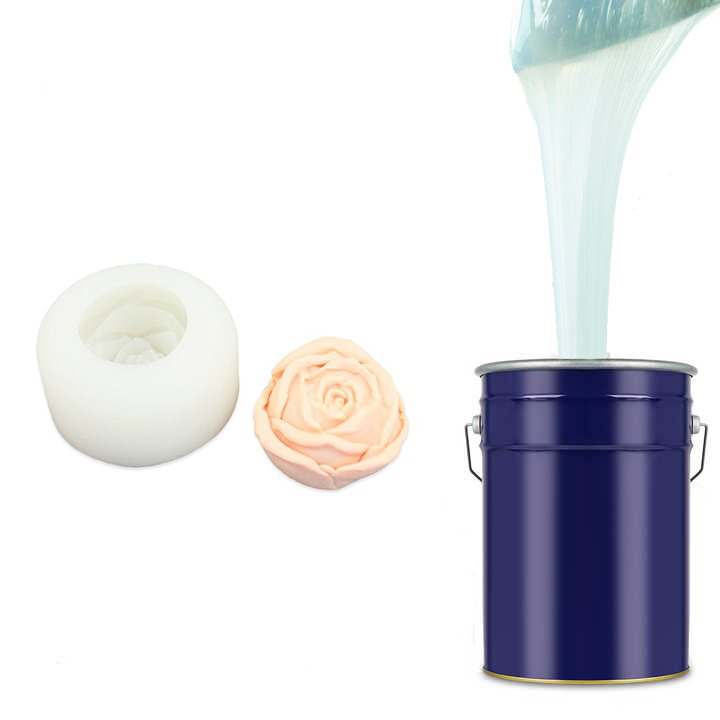
Mould In Silicone Eye (Eye) ISSN 1476-5454 (online) ISSN 0950-222X (print)