Thank you for visiting nature.com. You are using a browser version with limited support for CSS. To obtain the best experience, we recommend you use a more up to date browser (or turn off compatibility mode in Internet Explorer). In the meantime, to ensure continued support, we are displaying the site without styles and JavaScript.
Scientific Reports volume 14, Article number: 8368 (2024 ) Cite this article Automatic Brick Laying Machine
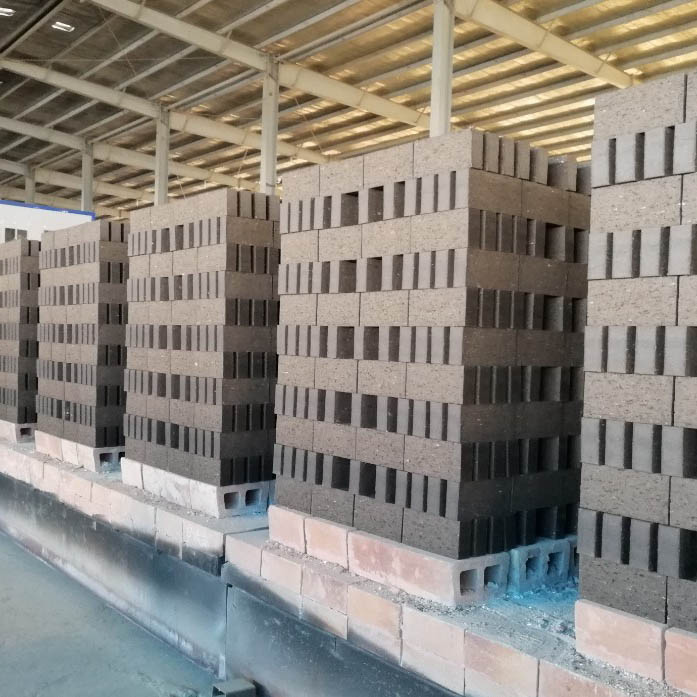
The exhaust gases in production of burnt clay bricks is responsible for greenhouse gases (GHGs) emission which increase the carbon footprint in the ecosystem. Here, we report carbon emission and thermal performance based evaluation of 8 ft. × 9 ft. × 8 ft. building. The bricks used in building construction are manufactured from fly ash, agro-forestry wastes, construction & demolition wastes (C&D), ground granulated blast furnace slag (GGBFS) using NaOH as activator in order to provide compressive strength in the range of 3–6 MPa with ambient curing at 30 °C for 28 days. Life cycle analysis (LCA) reveals the total CO2 emission for fly ash and burnt clay bricks estimated to be 43.28 gCO2 and 290 gCO2 per brick, respectively. Considering the current scenario, by replacing 1–2% of brunt clay bricks with agro-forestry waste, C&D waste based fly ash bricks can potentially reduce 0.5–1.5 million tons of CO2 emission annually. The embodied energy calculation shows fly ash based bricks consumes 10–15 times less energy as compared to burnt clay bricks. Thermal paremeters viz., U-value (0.5–1.2 W/m2K), thermal conductivity (0.4–0.5 W/mK) show adequate insulation of agro-forestry waste based fly ash bricks highlighting its importance of thermal comfort, CO2 reduction along with sustainable and eco-friendly construction practices.
Production of 1-burnt clay brick and 1-ton of cement results in approximately 0.41 kg and 0.85 ton of CO2 emission1,2,3. Utilisation of farm soil in burnt clay bricks reduces the fertility of agricultural soil, mining of natural aggregates, results in degradation and polluting river banks, poor air and agricultural land quality resulting in ecological imbalance. On an average, the fluoride concentration in soil is about 500 ppm which is released in the atmosphere from the brick kilns when fired at 1000 °C along with other harmful gases viz., CO2, SO2 and NOx posing threat to human health with acute respiratory problems4. The global production of clay bricks will reach to 1.5 trillion contributing to 20\(\%\) of global black carbon emission. As per literature, India’s current requirement of burnt clay bricks is approximately 200–250 billion bricks will soon rise to 700 billion bricks in infrastructure sector. According to world bank, the annual production of clay bricks in India is around 200 billion requiring 62 million tons of coal for its production. This will results in 41.6 million tons of CO2 emission5. Most of the brick manufacturing kilns are in northern part of India situated on the Indo-Gangetic plain comprising of fertile alluvial soil expanding over 700,000 km2 region. Approximately 60,000–70,000 brick kiln found in this region are fixed chimney bull’s trench kiln (FCBTK) which are old and traditional type kiln resulting in increased particulate matter and black carbon emission6,7,8.
Apart from the traditional building materials, waste generated from coal based power plants in the form of ash is also responsible for landfill and air pollution, and its proper utilisation is a huge challenge for energy and construction sector. According to a latest report by Ministry of Power, Govt. of India, the total fly ash generated form thermal power plants in India is approximately 270.82 million tons and out of which 259.86 million tons is utilised by various sectors with a utilisation of 95.95 percent9. The remaining fly ash may still cause serious land fill issues and land degradation problem if not properly utilised or disposed. The waste generated from construction and demolition activities in construction sector leads to degraded air quality with large amount of particulate matter (PM) and fine aerosols suspended in the atmosphere leading to respiratory problems in the vicinity areas. Between 2020 and 2021, major Indian cities have generated around 150 million tons of C&D waste10. Ground granulated blast furnace slag (GGBFS) which is a waste generated from iron and steel industry has shown a significant concern regarding its utilisation and proper disposal. According to the literature total amount of GGBFS production will reach around 270 million tonnes between 2020 and 2025 globally. In India, as per the study of Indian bureau of Mines, the annual GGBFS production is approximately be greater than 17 million tons with its major utilisation in slag based cements11,12,13.
Similarly, the wastes generated on the farm fields after crop harvesting is also a major issue in agricultural sector. In India, over 500 million tons of agricultural residue is generated annually14,15. The surplus agricultural residue which mostly accounts for rice and wheat, out of which 60% is burnt mostly at the farm fields16,17. The burning of these crop residues results in severe air pollution and poor air quality index in the nearby regions18. Similarly burning of forestry leaves also results in air pollution, fire hazards and black carbon emission.
Research on alkali-activated fly ash (AA-FA) with incorporation of various wastes as sustainable building materials has been done to address the waste management issues. Detailed insight on mechanical properties based on co-existence of sodium aluminosilicate gel (N–A–S–H) and calcium aluminosilicate hydrate (C–A–S–H) in presence of sodium silicate and NaOH solution of different molarities, with mechanical strength of 6–16 MPa have been reported by various researchers. Microstructural and mechanical properties of burnt clay bricks using C&D waste over the range of 0–100% have also been studied with compressive strength ranging from 5 to 15 MPa and thermal conductivity of 0.07–0.12 W/m K. Agricultural and industrial wastes-based bricks have been investigated in alkali activated fly ash by various researchers19,20,21,22,23. Various supplementary cementitious materials (SCMs) viz., fly ash (FA), GGBFS, silica fume (SF) utilisation in alkali activated concrete have shown to reduce GHGs emission and landfill problems. It is reported that addition of these SCMs in cement can reduced 13–22% of CO2 emissions24,25. However, the incorporation of treated/untreated agro-waste straw fibres, dried forestry leaves along with C&D wastes in construction materials has been scarcely reported. The effect of agro-forestry content viz., rice straw, dried forestry leaves with C&D waste and GGBFS addition on mechanical, thermal, acoustical and fire related properties of alkali activated fly ash bricks have been done in detail in the authors’ previous study26. The study has shown promising results of agro-forestry waste addition along with slag and C&D waste in fly ash bricks with 5 M alkali solution with compressive strength of 8–15 MPa, acoustic isolation of 35–50 dB and thermal conductivity of 0.4–0.5 W/m K.
The manufacturing of construction materials viz., bricks, cement and concrete etc., result in greenhouse gases (GHGs) emission causing a major disturbance in the ecosystem with rising global temperature leading to climate change. To evaluate the CO2 emission at various stages of a construction material production, life cycle assessment (LCA) is most widely used approach for determining the overall environmental impact27,28. LCA is a popular and rigorous method for assessing the overall environmental impact of building materials over its complete life cycle. The CO2 emission at various stages viz., extraction of materials, chemicals (NaOH) production resulting in 1.915 ton-CO2 emission per ton production, transportation of materials, fuel requirement, brick manufacturing, firing/treatment and storage/distribution steps must be considered while calculating the total CO2 emissions and over all environmental assessment29.
Apart from the CO2 emissions caused by building material production, providing thermal comfort to a building with external aids is also responsible for greenhouse gases (GHGs) emission. Improvement in energy efficiency of the building for optimum energy consumption in context with thermal comfort plays an important role in reducing the GHGs emission. In a study on dynamic thermal performance of conventional and alternative building wall envelopes provides a good insight regarding steady and dynamic state analysis of various building materials viz., porous autoclaved aerated, fly ash gypsum based bricks, conventional burnt clay bricks etc., and their respective building envelopes30. Authors’ previous study on dynamic and steady state thermal analysis on cement fibreboard and bamboo based buildings has elaborated parameters viz., U-value, thermal resistance, decrement factor, time lag and thermal admittance properties in detail31.
The present study explores various agricultural and industrial waste viz., fly ash, GGBFS, rice straw, forestry leaves and C&D waste based bricks in building construction. Investigations regarding life cycle assessment involving CO2 emission, embodied energy and thermal performance behaviour have been done in detail to provide a holistic framework for further research in the field of sustainable building products. In the present study, the authors report to minimise the CO2 emission by utilisation of fly ash, C&D waste and agro-forestry waste in construction of 8 ft. × 9 ft. × 8 ft. masonry building and compared with burnt clay bricks building. A study on CO2 emissions caused by agro-forestry, C&D waste based fly ash bricks and its comparison with burnt clay bricks, embodied energy calculation along with thermal (building envelope) analysis are reported and discussed in detail. The present work may be considered as the continuation of authors’ previous work done on properties evaluation of agro-forestry waste and C&D waste based fly ash bricks26.
The main objectives in the current study may be defined as:
Estimation of carbon emission, embodied energy of fly ash and burnt clay bricks.
Estimation of steady state parameters for agro-forestry waste, C&D waste based fly ash bricks and burnt clay bricks.
Evaluation of dynamic responses viz., time lag, decrement factor, thermal damping and thermal admittance.
Alkali-activated fly ash (obtained from thermal power plant, NTPC Dadri, Uttar Pradesh, India) bricks incorporating a mixture of locally available agro-waste (rice straw) from farm fields, dried bamboo leaves, syzygium cumini, mangifera indica as forestry waste mixed in equal proportion by weight percent in crushed form, GGBFS from Tata Steel Sahibabad, Uttar Pradesh, India, were used as raw materials. C&D waste from local demolition site consisting of red bricks, mortar, and concrete components as the constituents of fly ash bricks has been investigated in the present study. The fly ash collected from the thermal power plants is usually obtained from burning bituminous and anthracite coals which have high ash content (30–40%) and low calcium content (1–5%), classified as class ‘F’ fly ash characterised by x-ray fluorescence spectroscopy (XRF) method (Bruker S4 Poinner). 3 M sodium hydroxide (NaOH > 98%, Thomas Baker) solution with water to binder ratio of 0.4–0.5 was employed for brick casting.
The agro-forestry wastes were dried under ambient sunlight to remove excess moisture (up to 5%) that may cause any fungal or biodegradation of the stockpile and chopped to desired 5 mm of length of 3–5 mm thickness. The chemical compositions of fly ash which includes crystalline phases of quartz (SiO2), mullite (Al2O3), and hematite (Fe2O3) as major components and CaO, K2O, TiO2, MgO and MnO as minor components. Chemical composition of fly ash and GGBFS with physical properties viz., fineness, particle size have been mentioned in detail in Table 1.
Bricks were produced by a indigenously developed brick-making machine based on vibro-compaction technique employing a four-bar slider-crank linkage configuration based ejection mechanism for demolding, and a plunger weight of 15 kg for compaction with the options of 4–8 bricks per batch33. The dimension of the casted bricks was 230 mm × 110 mm × 70 mm. The raw materials utilised in brick casting are agro-forestry waste 2 wt% (of fly ash), GGBFS: 10 wt%, C&D waste: 15–20 wt% with approximate size of 50–80 mm × 40 mm × 40 mm with 3 M NaOH as activator solution. The ratio of fly ash to fine aggregate (sand) was in the ratio of 1:2. For comparison purpose, cement based (OPC-43 grade) and bottom ash bricks were also casted and tested for mechanical properties. The casted bricks were cured at ambient temperatures of around 30–32 °C for 28 days. The various steps involved for brick casting process are shown in Fig. 1. The nomenclatures of the investigated bricks are: A- cement based bricks, B- fly ash based bricks, C- bottom ash based bricks. The bottom ash collected from the same source has similar chemical constituents with a majority part of unburnt carbon residue/soot (black colour), which is a distinguished feature of bottom ash in physical appearance which was also visible in casted brick samples. The mix design parameters of the casted fly ash, cement and bottom ash bricks are shown in Table 2.
Process methodology for agro-forestry waste based fly ash, bottom ash and cement based bricks using vibro-compaction technique.
The elemental, compositional and quantitative analysis of fly ash, GGBFS, rice straw and forestry leaves have been mentioned in Table 1 and detailed study in the authors’ previous publications26,32 . The mechanical performance of the alkali activated fly ash (AA-FA) bricks was analysed by compressive strength test in accordance with relevant Indian standard codes34,35,36. The brick specimens have been tested on a universal testing machine (UTM) of 100-ton capacity (Shimadzu) with a loading rate of 140 kg/cm2/min as specified in IS code36.
A prototype building comprising of single room was constructed from agro-forestry waste, C&D waste based fly ash based bricks with dimensions 8 ft. × 9 ft. × 8 ft. to investigate the feasibility of bricks in non-load bearing application and evaluation of thermal and carbon emission parameters. The foundation of the building was 1 ft. × 1 ft. in width and depth laid on burnt clay bricks to provide additional strength to the foundation. The prototype building was fitted with a 3 ft. × 4 ft. double-glazed glass window and 6 ft. × 2.5 ft. door with a metallic sheet roof. The whole construction process starting from foundation to roof installation is shown in Fig. 2. A total of 1000 bricks comprised of 1000 kg of fly ash, 2000 kg of fine aggregate (sand), 150–200 kg of C&D waste, 20–25 kg of agro-forestry waste, and 100 kg of GGBFS as waste material was utilised in construction of prototype building.
Foundation laying and construction of fly ash based bricks building.
The environmental concern of developed fly ash bricks in terms of CO2 emission and its comparison with burnt clay bricks has been done in the present study by LCA approach analytically for cradle to gate cycle assessment. The calculations and analysis have included the resources and processes involved in manufacturing of the bricks. The various stages starting from extraction, transportation of raw materials to factory site, firing/casting, storage and distribution causing CO2 emission has been taken into consideration in the present study. Basic CO2 emission calculations for manufacturing burnt clay and fly ash bricks at various stages have also been discussed in subsequent sections.
The various stages in LCA analysis of burnt clay bricks include extraction of raw materials, manufacturing/firing, and storage/distribution.
In Indian scenario, the excavation and extraction of soil for brick manufacturing is labour intensive. According to the specification laid for the assessment of the greenhouse gases emission of goods and services of human energy involvement is excluded. Therefore, ‘soil excavation’ process is not included in CO2 emission and hence no estimation is required37.
The calculation of carbon emission involved in material transportation, is done by the “ton-kilometer” (measured in t-km) estimation. The ton-kilometer is the measurement for the transportation of 1-ton of a material by a given transport mode over a distance of 1 km. Subsequently, the CO2 emission due to transportation is then calculated by multiplying the value of t-km by the emission factor (in kgCO2/t-km) and the total mass of material transported38,39.
In Indian scenario, usually the clay field and the brick kiln is situated within the distance of 1–2 km. For 1-kg of brick manufacturing, ~ 1.11 kg of clay is required. Therefore, the t-km estimation for 2-km distance is
The average gCO2 emission of 40-ton truck/tractor is 62 g CO2/t-km38. By multiplying the above value with the gCO2 emission factor of 40-ton truck/tractor, the total CO2 emission per kg of brick is obtained as:
The transported clay which has been brought to manufacturing site situated near brick kiln location is kept and stored in pile form. The stored clay does not require any further additives prior to casting. The water requirement for clay brick casting is around 20–30%, usually taken from rivers, ponds or bore well pumped by 2–3 hp motor. On an average, the electrical power and CO2 emission factor associated with the electricity generation and transmission for desired amount of water for 100,000 bricks casting is around 18.77 kWh, 1.36 kgCO2/kWh and 0.47 kg CO2/kWh respectively40.
For 1-brick, the CO2 emission due to water requirement is given as
Post green brick casting, the bricks are sundried for about 10–15 days prior to firing in a kiln. Majority of the brick kilns are coal and bagasse fired. The firing process is a major cause of CO2 emission in clay bricks production.
The transportation of coal as fuel in brick kilns is done via. Rail routes in India from coal mines/fields. Then it is locally distributed to the nearby regions of brick kilns via road transports.
According to the literature38,39, the average CO2 emission per ton-km for coal transportation from an electric locomotive goods trains is 22 gCO2 per t-km.
Average ton-km calculation for 70 gm coal transported to a distance of 1000 km is:
Therefore, the average CO2 emission for fuel transport via rail route is:
Ton-km estimation for coal as fuel transport from railway wagons to local kilns situated at a distance of 50 km is:
Average gCO2 emission of 40-ton truck/tractor for coal transportation is given as:
The CO2 emission from coal firing for 1-kg of brick is ~ 64.26 gCO2. During the firing of bricks, the calcium carbonate (CaCO3) present in the clay decomposes into calcium oxide (CaO) and CO2 resulting in emission of 25.9 gCO2/kg of fired brick40,41.
After firing process, the bricks are stored in the vicinity areas for storage and distribution. For a particular kiln site, the distribution area is situated within the range of 25–30 km.
The ton-km estimation of transporting 1 kg of brick to a distance of 30 km can be calculated as:
The average gCO2 emission of 40-ton truck/tractor is 62 g CO2/ton-km. the total CO2 emission per kg of brick distribution to a distance of 30 km is obtained as:
For total CO2 emission estimation, overall CO2 emissions due to all the operations involved in the burnt clay brick manufacturing can be summarized as:
The CO2 emission norms from mini diesel mini in India, according to international council on clean transportation is 143.1 gCO2/km, with load carrying capacity of 1 ton and 0.011 gCO2/t-km for electric locomotive (freight trains) in India42,43.
In the present study, fly ash as raw material is transported from Dadri power plant (Uttar Pradesh, India). The ton-km estimation for 180 km distance and 1 kg of fly ash is given as
By multiplying the above value with the emission of 40-ton truck, the total CO2 emission per kg of fly ash is obtained as:
The CO2 emission due NaOH production results in 1.915 ton-CO2 emission per ton (of NaOH produced). For 3 M solution used in brick casting with water: binder ratio of 0.3, the emission due to NaOH utilisation is around 20.56 gCO2. The emission due to GGBFS utilisation may be considered to 0.52 gCO2.
The water requirement for agro-forestry and C&D waste fly ash bricks in the present study is in the range of 0.4–0.5. The CO2 emission due to water pumping (required for 1-kg of brick) is taken similar to the clay brick which is given as:
The C&D waste generated from demolition of buildings, structures etc., requires a diesel powered equipment. The approximate value of energy consumption is 0.0359 MJ of diesel per kg of brick demolished. The energy content of diesel is 38.29 kJ/litre44. The energy required for 1-kg of brick demolition is 35.9 kJ which consumes 0.93 L of diesel. The C&D waste generated from the site is transferred to the brick casting site within 2 km range and crushed manually to the desired size in the present study.
The ton-km estimation for transporting 1-kg of C&D waste to a distance of 2 km is given as
The average gCO2 emission of 40-ton truck to carry 1 kg of C&D waste as described by:
1-brick utilises approximately, 150–200 gm of coarse C&D waste, therefore net CO2 emission will be 0.024 \({{\text{gCO}}}_{2}\) .
The transportation of agro-forestry wastes is usually done within 10 km range. The ton-km estimation is given as
The average gCO2 emission of 40-ton truck to carry 1 kg of agro-forestry waste is:
1-brick utilises approximately, 10 gm of agro-forestry waste, therefore net CO2 emission will be 0.6E-6 \({{\text{gCO}}}_{2}\) .
The fine aggregate (sand) is transported from nearby river bank situated around 80 km from the casting site. The ton-km estimation is given by:
The average CO2 emission per kg of material by 40-ton truck is given as:
1-brick utilises 2 kg of fine aggregate, therefore net CO2 emission will be 9.92 \({{\text{gCO}}}_{2}\) .
The electricity requirement for brick manufacturing by the indigenous brick making machine utilizing 3 hp motor is ~ 458 kWh (for 100,000 bricks, vibration and loading for 30 s) resulting in CO2 emission 1.36 kg CO2/kWh and 0.47 kg CO2/kWh for generation and transmission, respectively.
The CO2 emission for electricity generation and transmission per kWh is 1.83 gCO2.
Therefore, the CO2 emission for 100,000 brick casting using electric brick making machine is given by:
Therefore, 01 brick casting would result in 0.008 gCO2 emission.
The casted bricks are dried/cured at ambient temperature for 28 days for optimum strength gain and does not require any firing process.
The ton-kilometer estimation of transporting 1 kg of brick to a distance of 30 km, can be calculated as:
The total CO2 emission per kg of brick distribution to a distance of 30 km is obtained as:
1-brick weighs approximately, 3–3.2 kg, therefore net CO2 emission will be 5.95 \({{\text{gCO}}}_{2}\) .
For total estimation of carbon emission by fly ash bricks, total CO2 emissions due to all the operations involved in the fly ash bricks production can be summarised as:
Embodied energy calculation in the present study have been done using LCA approach along with inventory for carbon and energy database. The embodied energy may be defined as total primary energy (e.g., coal; energy extracted form nature) utilised from direct and indirect processes associated with a product or services within the boundaries of cradle-to-gate. This boundary approach includes the manufacturing process starting from raw material extraction, transportation and manufacturing until the end product is ready to leave the factory gate45. Embodied energy has a great importance in the context of buildings and construction materials because a high energy intensive material is more likely to cause larger carbon emission.
The thermo-physical properties viz., thermal conductivity (k), specific heat capacity (Cp) and density (ρ) are basic thermal properties of any building materials. The thermal properties derived from the basic properties are thermal transmittance (U), thermal diffusivity (α), thermal effusivity (τ), and thermal mass (m) are equally important for thermal properties evaluation. Steady-state thermal properties of building envelope were calculated according to Indian standard IS 3792–197846.
The constructed agro-forestry waste based fly ash bricks and burnt clay brick walls of the respective buildings were tested for temperature variations on inner and outer wall surfaces, ambient and inside room temperature for a period of 24 h and 07 days for June month. The instrument used for wall temperature measurement was an infrared thermometer Fluke 64 MAX having a temperature range of − 30 °C to 600 °C with an accuracy of ± 1.5 °C. According to guide for heat insulation of non-industrial buildings IS 3792–1978, no heating or cooling aids were used during the measurement. The thermal transmittance (U-value) of fly ash brick wall and burnt clay brick wall envelope was measured by Testo 635 U-value probe meter based on the formula:
where, α is 7.69 W/m2K, Tin inside room temperature, Tsurface, in is inside room surface temperature, Tout is outside ambient temperature.
Non-steady state thermal properties viz., time lag, decrement factor, thermal damping and thermal admittance are dynamic thermal properties which provide real time assessment of building envelopes. In this study, the thermal performance of building walls has been investigated by admittance method.
Authors’ previous study has explored the utilisation of alkali-enzyme treated agro-forestry residues along with C&D waste in fly ash bricks26. In the present study, a simple approach has been adopted for agro-forestry waste utilisation. The mixture of untreated rice straw and dried forestry leaves (2 wt% of fly ash) crushed and chopped to desired size mixed in fly ash and sand along with C&D waste. Figure 3a,b show the density and compressive strength values of 2-wt% added agro-forestry waste and C&D waste fly ash bricks along with bottom ash and cement based bricks. The cement and bottom ash based bricks investigated in the present study are only for comparison purpose. The density of these bricks shows a reducing trend with values ranging from 1400 to 1600 kg/m3 as shown in Fig. 3a. The compressive strength of the bricks lies in the range of 1.5–8 MPa with lowest value corresponding to bottom ash bricks and 3–4 MPa for fly ash based bricks and maximum for cement based bricks shown in Fig. 3b. The cement based bricks have highest density and compressive strength due to better formation of hydration products viz., calcium silicate hydrate (C–S–H).
Mechanical aspects of agro-forestry waste based cement, fly ash and bottom ash bricks (a) Density and (b) Compressive strength variation of bricks for curing of 28 days, (c) CO2 emissions analysis.
For fly ash based bricks, the reduction in compressive strength as compared to authors’ previous study may be attributed to low degree of formation of hydration products viz., sodium aluminate silicate hydrate (N-A-S–H) or calcium/sodium aluminate silicate hydrate (Ca–Na)–A–S–H gel. The reduced formation of the hydration products may be attributed to low molarity of NaOH solution (3 M) which results in lesser dissolution of aluminosilicates to release SiO4 and AlO4 tetrahedral units responsible for polycondensation process. The addition of GGBFS in the mix design partially substitutes Na ions with Ca ions resulting in formation of (Ca–Na)–A–S–H gel with quick hardening of the mix at ambient temperatures47,48. The authors’ detailed previous study has shown the effect of slag addition, NaOH molar concentration, average particle size on physical properties viz., compressive strength, density, porosity with varying slag content in fly ash bricks26. The bottom ash bricks show poor compressive strength due to the presence unburnt carbon as agglomerates resulting in coarser particles (~ 150 µm) which limits the reactivity of alkaline solution and dissolution of aluminosilicates in the mix. The bottom ash absorbs most of the alkali solution, which increases the liquid to solid ratio with reduced reactivity. The quality of the bottom ash can be further improved by mechanical activation process, through combustion to reduce the LoI/unburnt carbon content which will result in enhanced reactivity. But the process of making bottom ash reactive is energy intensive and has environmental issues related to carbon emission49.
The particle size and shape of slag and fly ash play an important role as the two parameters are responsible in determining the chemical reactivity leading to enhanced physical parameters. The fly ash utilised from Dadri power plant and GGBFS have an average particle size of 25.6 µm and 25.1 µm respectively. The smaller particle size results in higher surface area which increases the overall reactivity at solid–liquid interface. According to the literature, the smaller size particles have greater amorphous content as they quench more quickly than larger particles50. The x-ray photoelectron spectroscopy (XPS) analysis of the various fly ash done in the authors’ previous study has able to identify the major constituents and their amorphous phases present in the fly ash samples. The amorphous (reactive) content of silica present in fly ash responsible for reactivity with alkali solution resulting in polycondensation process has been studied in detail32. Furthermore, the spherical shape of particles improves the workability of the mix at lower liquid to binder ratio resulting in less water requirement. The thermal conductivity data for 25 mm thick agro-forestry based fly ash sample shows the value over the range of 0.4–0.45 W/m K26. The burnt clay brick sample has the thermal conductivity value of 0.8 W/m K, which is almost double the value of fly ash brick samples. The enhanced value of thermal insulation of fly ash brick samples will provide better thermal insulation and comfort to the building occupants. A brief carbon emission analysis of cement, fly ash and bottom ash bricks is shown in Fig. 3c. From the graph 3(c), it is evident that even fly ash bricks with alkali activation shows moderate CO2 emission as compared to cement based bricks. This is attributed to high CO2 emission associated with NaOH production which is nearly 1.915 ton-CO2/ton NaOH produced. The overall emission factors have been taken from Table 3 regarding brick manufacturing.
Post mechanical analysis of fly ash bricks, the environmental impact analysis of fly ash bricks by LCA approach and its comparison with burnt clay bricks has been investigated in this section. The various stages in LCA analysis of both the type of bricks include extraction of raw materials, manufacturing/firing, distribution, demolition, and end of life cycle. A diagram showing the system boundary for process illustration for burnt clay and fly ash bricks production is shown in Fig. 4. The diagram shows outer enclosed structure as system boundary and red line in interior part as production stage of the bricks.
System boundary conditions for (a) Burnt clay bricks and (b) Fly ash bricks. Outer enclosed structure shows system boundary (blue colour) and inner enclosed structure shows production stage (red colour).
For total estimation of CO2 emission for burnt clay bricks, overall CO2 emissions due to all the operations involved in the burnt clay brick manufacturing as mentioned in detail in Section "CO2 emission analysis by LCA approach and embodied energy estimation" is considered. According to Eq. (10), the overall carbon emission values for 1 kg of brick production is 97.08 gCO2 and 270 gCO2 per brick according to European emission values38,39.
The CO2 emission from diesel mini trucks in India, according to international council on clean transportation is 143.1 gCO2/km, with load carrying capacity of 1 ton and 0.011 gCO2/t-km for electric locomotive (freight trains) in India42,43.
By substituting the above emission values, the overall CO2 emission of burnt clay bricks manufactured according to Indian emission norms is approximately 102 gCO2 per kg of clay brick.
Average weight of 1 burnt clay brick is ~ 2.8 kg. Therefore, total \({{\text{CO}}}_{2}\) emission for 1 brick ~ 285.6 gCO2 according to Indian transport emissions of vehicles and freight trains. The complete CO2 emission in burnt clay bricks production is summarised in Table 3.
For total estimation of carbon emission by fly ash bricks, total CO2 emissions due to all the stages considered in fly ash bricks production can be calculated according to Eq. (23) as 43.28 gCO2 per brick. The total CO2 emission for 1000 fly ash bricks is 38.26 kgCO2 in construction of prototype single room masonry building in the present study.
The CO2 emission from diesel mini trucks per km in India, according to ICCT is 143.1 g CO2/km, considering load carrying capacity of 1-ton42. The values of carbon emission either by passenger or goods vehicles vary because there is no official breakdown of road fuel use data by various vehicle types in India. For majority of Asian countries, there are also no published data on vehicle-km or ton-km for goods vehicle by the main modes of transportation. Therefore, it is not possible to estimate CO2 emissions for each major activity within the transport sector51. By putting the above emission values, the overall CO2 emission of agro-forestry waste based fly ash bricks manufactured according to Indian emission norms is approximately 27.63 gCO2 per kg of brick. Weight of 1 fly ash brick is ~ 3.0 kg. Therefore, total emission for 1 brick as per Indian emission values ~ 82.89 gCO2.
The CO2 emission values at various stages for burnt clay and fly ash bricks with both (European and Indian) emission values is summarised in Table 3. The CO2 emission from major steps and their percentage distribution of fly ash and burnt clay bricks is shown in Fig. 5c,d. Approximately, 90% of carbon emission in fly ash brick production arises from material transportation and demolition activities (Fig. 5a) and 10 percent from distribution activity. Similarly, for burnt clay bricks production, the major portion (around 92%) of carbon emission is due to firing process and carbonates decomposition of carbonates present in the clay/soil (Fig. 5b).
Carbon emission at various stages of bricks production. Percentage wise CO2 emission for (a) Fly ash and (b) Burnt clay bricks, (c) and (d) CO2 emission from at various production stages of fly ash and burnt clay bricks.
Net CO2 reduction of utilizing agro-forestry waste and C&D waste based fly ash bricks when compared with burnt clay bricks is ~ 246 gCO2 per brick as per European emission norms. According to a literature study, the overall CO2 emissions in manufacturing of burnt clay bricks in clamps is around 195 gCO2/kg of fired brick40. In the present study, the overall CO2 emission for burnt clay bricks is around 102 gCO2/kg of brick calculated with Indian emission values. From Table 3, it is evident that carbon emission values of burnt clay bricks are almost 4–8 times higher than carbon emission of fly ash bricks with a similar trend in embodied energy values described in next section. A similar study on utilisation of boiler ash collected from pulp and paper mills with alkali activation in masonry construction materials has been reported. The obtained ash mixed with clay and lime activated with 2 M NaOH solution exhibits compressive strength in the range of 11–16 MPa cured at 30 °C for 28 days. The compressive strength of fly ash bricks with 3 M NaOH activation in the present study lies in range of 3–6 MPa. The environmental impact assessment of boiler ash shows Ca(OH)2 and NaOH as biggest CO2 emitters (30–65% of total emission) as their production is energy intensive and 90% of CO2 emission for burnt clay bricks during firing process. The values reported in the literature is in agreement with the carbon emission values obtained in the present study. Another similar study regarding environmental assessment on rice husk ash incorporation in fly ash bricks with alkali activation shows almost 60–90% of environmental impact is due to manufacturing of raw materials49,52.
In the present study, the embodied energy of a typical building/construction materials may be defined as overall primary energy consumed starting from raw material extraction, manufacturing, storage and transportation till the material is ready for distribution at the factory gate. This type of approach is usually termed as cradle to gate approach which is employed in the embodied energy estimation. Table 4 shows the embodied energy of both burnt clay bricks and fly ash bricks constituents and total energy consumed45. From the estimation it is clear that burnt clay bricks are 10–15 times more energy intensive compared to fly ash bricks which consumes more energy during the firing process resulting in CO2 emission as evident from LCA analysis done in previous section.
Considering the current requirement of clay bricks in India which is around 250 billion bricks per year, the overall carbon emission values would be 72.5 billion tons CO2. According to a report published by CEA (2022), the total utilisation of fly ash in manufacturing of bricks and tiles is 31.62 million tons which is 11.68% of total fly ash utilisation and 25.41% is utilised by various cement industries. Out of total fly ash generation of 270.82 million tons from thermal power plants, still approximately 11 million tons of fly ash is non-utilised9. The extraction of top fertile soil for burnt clay brick production causes land degradation resulting in reduced soil carbon content, microbial activity and nitrous oxide concentrations. The consequence of this activity may alter the CO2/GHGs concentration in the atmosphere to a great extent with increase in global temperature and change in weather patterns. Whereas, the fly ash with properties viz., odorless, non-toxicity, non-flammability with minimum threat to human health may serve as potential substitute for burnt clay bricks. Fly ash based bricks have low water permeability, shrinkage, enhanced workability, high thermal insulation and chemical resistance towards sulphates and chlorides and excellent finishing. All these features of fly ash based bricks makes it economical and a potential green building material along with other incorporated wastes. The cost of the fly ash bricks varies with many parameters viz., material cost, quality, transportation charges and manpower charges. In the present case, the cost of one fly ash brick is approximately ₹3–6 considering all the costs including material transportation and electricity consumption. The price of one burnt clay brick also varies from region to region with similar factors as mentioned for fly ash bricks.
After mechanical, thermal and LCA analysis, a brief investigation of moisture and algae growth on fly ash brick and burnt clay brick building has been done during monsoon to assess the suitability of fly ash bricks for outdoor application. Figure 6 shows the exterior walls of both the buildings exposed to seasonal rainfall (monsoon period) for the period of June to July. The average moisture content of agro-forestry waste based fly ash brick building is 25–40%. Whereas, the moisture content for burnt clay brick building lies in the range of 40–50% which shows higher water absorption of burnt clay bricks. The exterior walls of both the buildings for the summer season is also shown in Fig. 6a, where no algae or fungal growth are observed. From Fig. 6b, algae and fungal growth during monsoon season on external brick wall of burnt clay brick building can be clearly seen which may lead to deterioration of wall surfaces. Whereas, no degradation or visual damages are observed for fly ash brick building due to their low water absorption and chemically (alkali) treated bricks.
Physical appearance of fly ash and burnt clay bricks building during (a) Summer season, (b) Monsoon (rainy) season.
The aim of studying thermal properties of building materials is to investigate the indoor performance and thermal comfort of the buildings. Steady state parameters viz., thermal conductivity, thermal transmittance (U-value), thermal diffusivity, specific heat and thermal effusivity are determined and compared for fly ash and burnt clay bricks. The investigated building walls are shown schematically in Fig. 7 and wall dimensions, properties are mentioned in Table 5.
Schematic wall configurations of fly ash and burnt clay brick walls.
The one dimensional (1-D) heat transfer process may be written as:
where, \(\lambda\) is the thermal conductivity, ρ is the density, and cp is the specific heat capacity of the building wall materials.
The Eq. 25 can be rewritten as:
where, \(\alpha\) is the thermal diffusivity defined as (\(\lambda\) /ρ. Cp) and expressed in m2/s. The thermal diffusivity may be defined as rate at which thermal disturbance propagates and is a thermo-physical property of the material.
The thermal effusivity which is ability of material to exchange heat with the surroundings can be written as,
where as, \(\xi\) is thermal effusivity in J m−2 K−1 s0.5, ρ is the density of the material, Cp is specific heat capacity in J Kg−1 K−1.
Thermal mass or volumetric heat capacity which is defined as \(m= \rho . c\) is the ability to store thermal energy.
Considering the density of 1400 and 1650 kg/m3 and specific heat capacity of 930 and 880 J/kg K for fly ash and burnt clay bricks respectively, the various steady state parameters for both the bricks are calculated and shown in Table 6.
As evident from the Table 6, the fly ash bricks have lower thermal mass (\(m\) ), lower U-value, thermal diffusivity (\(\alpha\) ) and effusivity (\(\xi\) ) as compared with burnt clay bricks revealing better thermal insulation and low thermal energy storage capacity. Walls with lower U-value shows a better performance to indoor surface temperature variations. Figure 8 shows outer and inner wall temperature variations for different directions of the fly ash brick building. The maximum observed temperature for outer surface walls varies over the range of 38–48 °C and minimum temperature of 28–30 °C. Whereas, the inner maximum wall temperature varies over the range of 36–40 °C and minimum temperature of 29–32 °C, recorded for 24 h period. The higher inner wall temperature is attributed due to metallic sheet roof and double glazed window on west facing wall which causes the entrapment of heat energy due to non-ventilated condition of the building. The outer and inner wall temperature variations are shown in Fig. 9 for different directions of the burn clay brick building. The maximum observed temperature for outer surface walls varies over the range of 40–46 °C and minimum temperature of 29–30 °C. Whereas, the inner maximum wall temperature varies from 36 to 38 °C and minimum temperature between 29 and 31 °C, observed for 24 h. The lower inner wall temperature fluctuations are attributed to higher thermal mass and subsequently higher thermal admittance of the burnt clay bricks over fly ash bricks described in next section.
Wall temperature variation of agro-forestry waste based fly ash brick wall envelope.
Wall temperature variation of burnt clay brick wall envelope.
Non-steady state i.e., dynamic calculations allow the thermal performance of the buildings in real time condition. The temperature variations of the external/outside environment have a profound effect on building envelope and dynamic properties viz., time lag, decrement factor, thermal damping, and thermal admittance30,53,54. Thermal admittance of a material (Y) can be defined as ability to absorb heat energy and release it over a period of time after the removal of heat source. This is an indicator of the thermal storage capacity (thermal mass) of a building or wall material. Thermal admittance is expressed in W/(m2K), where the higher the admittance value, larger the thermal storage capacity of the wall material. The large value of thermal admittance (Y) indicates lower temperature fluctuations inside the buildings. Typical admittance values are based on a 24-h temperature cycle. It measures the heat flow rate entering the internal surface of a wall as a response to a unit cyclic temperature fluctuation. The thermal admittance of both the buildings have been calculated by the transmission matrix method.
Time lag and decrement factors are critical thermal performance characteristics that influence the heat storage capabilities of any materials. Time lag (Φ) is the time difference between the maximum temperature at the outer and inner walls with periodic heat flow through the test specimen30,53.
The time lag can be written as:
where, \({t}_{{T}_{in\left(max\right)}}\) and \({t}_{{T}_{out\left(max\right)}}\) are the time of day when the inside and outside surface temperatures reach maximum value.
Another parameter i.e., decrement factor (\(DF\) ) may be defined as:
where, \({T}_{in({\text{max}})}\) , \({T}_{in({\text{min}})}\) are maximum and minimum inside wall temperatures and \({T}_{out({\text{max}})}\) , \({T}_{out({\text{min}})}\) are maximum and minimum outside wall temperatures.
Thermal damping is also an important characteristic based on the thermal resistance of the material (expressed in percent) which can be written as
where, \({T}_{o}\) and \({T}_{in}\) are maximum outside and inside wall temperatures, respectively.
For thermal admittance calculation, the transmission matrix \(Z\) of the multi-layered wall is obtained through the product of the matrices related to each layer, including the transmission matrix containing the film thermal resistances:
The thermal admittance function \(Y\) can be written as \(\frac{{Z}_{4}}{{Z}_{2}}\) (W/m2K), \(n\) is the number of homogeneous layers, Rso and Rsi are film thermal resistances54,55.
The elements of the transmission matrix can be written as:
where, i is the imaginary part, t is cyclic thickness and \(\xi\) is the thermal effusivity, \(L\) is the wall thickness, and P is the time period of the cyclic energy transfer. Both cyclic thickness and thermal effusivity can be expressed as:
\(\xi\) is thermal effusivity in J m−2 K−1 s0.5, ρ is the density of the material in kg m−3, c is specific heat capacity in J Kg−1 K−1 and P is the number of period hours.
The outside and inside surface temperatures of fly ash and burnt clay brick building walls for all the directional faces are shown in Figs. 8 and 9 respectively. The various parameters viz., time lag, decrement factor, thermal admittance and thermal damping have been calculated and listed in Table 7.
As evident from Table 7, the higher time lag, lower decrement factor and high thermal damping and large thermal admittance parameters are responsible for maintaining the indoor temperatures at nearly constant temperature for burnt clay brick building envelope. Overall, the steady state and dynamic state thermal analysis helps in understanding the performance behaviour of a building envelope and selection of materials as per the ambient weather requirement.
With the perspective of the current study, there are many possibilities and challenges regarding fly ash, agro-forestry waste and C&D waste utilisation in construction sector. The paramount challenge in agro-waste management is the lack of awareness among the farmer community towards environmental and health aspects of current and future generations. In recent times, the waste management and its utilisation in construction materials has shown a positive development which includes fly ash, C&D waste use in cement industry and construction sector. These utilisation of wastes reduces the burden on environment (excavation, processing) since most of construction materials viz., sand, aggregates and cement manufacturing relies heavily on natural resources. In the present study, an attempt to adopt the various wastes in building materials to reduce the environmental threat resulting in less carbon emission of the developed product as compared to conventional burnt clay bricks thus creating sustainable building products. According to an estimation, by replacing 1–2% of brunt clay bricks with agro-forestry waste, C&D waste based fly ash bricks can potentially reduce 0.5–1.5 million tons of CO2 emission. The thermal analysis of fly ash brick building has shown better thermal insulation as compared to burnt clay bricks which may provide better thermal comfort to the occupants. The future work related to the current study includes full scale fire testing of studied bricks in partition walls, sound isolation analysis and utilisation of the mentioned waste materials in additive manufacturing in construction. The practical applications of the present study involve creation of eco-friendly building products for sustainable construction practices promoting circular economy and waste to wealth creation.
The present work has demonstrated the potential utilisation of fly ash, C&D waste and agro-forestry waste as green building and environmental friendly materials. The mix design of the desired materials with 3 M NaOH activation leads to mechanical strength of 3–5 MPa within density range of 1400–1600 kg/m3. The lower compressive strength value of fly ash bricks is attributed to low molar (3 M) presence of NaOH activator resulting in lesser dissolution of aluminosilicates which releases SiO4 and AlO4 tetrahedral units responsible of promoting polycondensation process. The thermal conductivity ‘k’ values of 0.40–0.45 W/m K, of fly ash bricks results in better thermal insulation as compared to burnt clay bricks (‘k’: 0.8 W/m K) which may lead to thermal comfort for building occupants26.
The overall CO2 emission for manufacturing fly ash bricks in the present study is estimated at around 14.7 gCO2/kg of brick as compared to 97 gCO2/kg of burnt clay brick. The total CO2 emission from construction of prototype building with fly ash bricks is ~ 43.8 kgCO2. The net CO2 emission reduction for 1000 (utilised in prototype building construction) between fly ash and burnt clay bricks is ~ 245 kgCO2. Agro-forestry waste, C&D waste based fly ash bricks can potentially reduce 0.5–1.5 Mt of CO2 emission even if 1–2% of burnt clay bricks is replaced (and restricted from manufacturing) and used in construction. The embodied energy estimation shows the agro-forestry waste based fly ash bricks is 10–15 times less energy intensive than burnt clay bricks which supports the low carbon emission values of fly ash bricks.
Thermo-physical properties both steady and dynamic state viz., U-value, thermal conductivity, thermal diffusivity, thermal mass, time lag, decrement factor and thermal admittance etc., have been evaluated. The agro-forestry waste based fly ash bricks have shown lower values of U-value, thermal conductivity, thermal diffusivity, and high thermal mass, admittance and thermal damping for burnt clay bricks which are responsible in maintaining the indoor temperature variations with minimum fluctuations. These type of bricks may be utilised in hot weather environment for thermal comfort of the building occupants with energy savings. Considering the current demand of burnt clay bricks in India, the fly ash bricks along with agro-forestry waste, C&D waste has the potential to substitute burnt clay bricks in construction which can reduce the carbon footprint, land degradation and soil nutrients arising from burnt clay bricks production which is contributing to climate change and ecological imbalance and severe human health hazards.
All data generated or analysed during this study are included in this published article.
Inventory of Carbon and Energy
Fixed chimney bull’s trench kiln
Ground granulated blast furnace slag
Arezoumandi, M., Volz, J. S., Ortega, C. A. & Myers, J. J. Effect of total cementitious content on shear strength of high-volume fly ash concrete beams. Mater. Des. 46, 301–309 (2013).
Maraveas, C. Production of sustainable construction materials using agro-wastes. Materials 13, 262 (2020).
Article ADS CAS PubMed PubMed Central Google Scholar
Fiala, L., Konrád, P., Fořt, J., Keppert, M. & Černý, R. Application of ceramic waste in brick blocks with enhanced acoustic properties. J. Clean. Prod. 261, 121185 (2020).
Jha, S. K., Nayak, A. K., Sharma, Y. K., Mishra, V. K. & Sharma, D. K. Fluoride accumulation in soil and vegetation in the vicinity of brick fields. Bull. Environ. Contam. Toxicol. 80, 369–373 (2008).
Article CAS PubMed Google Scholar
Eil, A., Li, J., Baral, P., & Saikawa, E. Dirty Stacks, High Stakes. An Overview of Brick Sector in South Asia. A Report by World Bank (2020).
Agarwal, A., Overview on brick kiln: pollution, technology and where we need to go? (2015).
Akhtar, M. N. et al. Flyash-based bricks: An environmental savior—a critical review. J. Mater. Cycles Waste Manag. 24, 1663–1678 (2022).
Maity S., Alternate building materials- Brick Publisher: Development alternatives sector status Narain, S., Anil Agarwal Dialogue (New Delhi) (2015).
Central Electricity Authority (CEA), Report on fly ash generation at coal / lignite based thermal power stations and its utilization in the country for the year 2020 – 21, 1–86 (2021).
Tezeswi, T. P. & MVN, S. K. Implementing construction waste management in India: An extended theory of planned behaviour approach. Environ. Technol. Innovat. 27, 102401 (2022).
Blesson, S., Rao, A. U., Bhandary, R. P., Shetty, P. P. & Thomas, B. S. Comparative characteristics assessment of calcined and uncalcined agro-based waste ash with GGBS and its application in an alkali-activated binder system. Cogent Eng. 10, 2220483 (2023).
Blesson, S. & Rao, A. U. Agro-industrial-based wastes as supplementary cementitious or alkali-activated binder material: A comprehensive review. Innovat. Infrastruct. Solut. 8, 125 (2023).
Indian Minerals Yearbook, (Part- II: Metals and Alloys) 57th Edition; Slag – Iron and Steel. Government of India, Ministry of Mines, Indian Bureau of Mines (2018).
Mathur, R., & Srivastava, V. K. Crop residue burning: Effects on environment. Greenhouse Gas Emissions: Challenges, Technologies and Solutions, 127–140 (2019).
Ravindra, K., Singh, T. & Mor, S. Emissions of air pollutants from primary crop residue burning in India and their mitigation strategies for cleaner emissions. J. Clean. Prod. 208, 261–273 (2019).
Singh, J. Paddy and wheat stubble blazing in Haryana and Punjab states of India: A menace for environmental health. Environ. Quality Manag. 28, 47–53 (2018).
Bhuvaneshwari, S., Hettiarachchi, H. & Meegoda, J. N. Crop residue burning in India: policy challenges and potential solutions. Int. J. Environ. Res. Public Health 16, 832 (2019).
Article CAS PubMed PubMed Central Google Scholar
Bimbraw, A. S. Generation and impact of crop residue and its management. Curr. Agric. Res. J. 7, 304 (2019).
Dos Reis, G. S. et al. Fabrication, microstructure, and properties of fired clay bricks using construction and demolition waste sludge as the main additive. J. Clean. Prod. 258, 120733 (2020).
Priyadarshini, M., Giri, J. P. & Patnaik, M. Variability in the compressive strength of non-conventional bricks containing agro and industrial waste. Case Stud Const. Mater. 14, e00506 (2021).
Murmu, A. L. & Patel, A. Towards sustainable bricks production: An overview. Construct. Build. Mater. 165, 112–125 (2018).
Thakur, A. K., Pappu, A. & Thakur, V. K. Synthesis and characterization of new class of geopolymer hybrid composite materials from industrial wastes. J. Clean. Prod. 230, 11–20 (2019).
Raut, S. P., Ralegaonkar, R. V. & Mandavgane, S. A. Development of sustainable construction material using industrial and agricultural solid waste: A review of waste-create bricks. Construct. Build. Mater. 25, 4037–4042 (2011).
Wu, M. et al. Reducing environmental impacts and carbon emissions: Study of effects of superfine cement particles on blended cement containing high volume mineral admixtures. J. Clean. Prod. 196, 358–369 (2018).
Flower, D. J. M. & Sanjayan, J. G. Greenhouse gas emissions due to concrete manufacture. Int. J. Life Cycle Assess. 12, 282–288 (2007).
Singh, S. et al. Investigation of agro-forestry and construction demolition wastes in alkali-activated fly ash bricks as sustainable building materials. Waste Manag. 159, 114–124 (2023).
Article CAS PubMed Google Scholar
Kua, H. W. & Kamath, S. An attributional and consequential life cycle assessment of substituting concrete with bricks. J. Clean. Prod. 81, 190–200 (2014).
Koroneos, C. & Dompros, A. Environmental assessment of brick production in Greece. Build. Environ. 42, 2114–2123 (2007).
Alsalman, A., Assi, L. N., Kareem, R. S., Carter, K. & Ziehl, P. Energy and CO2 emission assessments of alkali-activated concrete and Ordinary Portland Cement concrete: A comparative analysis of different grades of concrete. Clean. Environ. Syst. 3, 100047 (2021).
Balaji, N. C., Mani, M. & Reddy, B. V. Dynamic thermal performance of conventional and alternative building wall envelopes. J. Build. Eng. 21, 373–395 (2019).
Singh, S., Chourasia, A., Bisht, R. S. & Yadav, S. Thermal and acoustic performance of cement fibreboard and bamboo buildings. Indian J. Eng. Mater. Sci. (IJEMS) 28, 462–471 (2021).
Singh, S. & Maiti, S. Investigation of glassy phase silica in low calcium content fly ash by X-ray photoelectron spectroscopy. Surface Interface Anal. 54, 45–51 (2022).
Bisht, R. S., Panigrahi, S. K., Singh, S., Kumar, D. & Yadav, S. Design analysis of a machine for manufacturing of bricks from industrial waste: Simulations and experiments. Int. J. Interactive Design Manufact. (IJIDeM) 15, 587–596 (2021).
IS 3495: Part 1, Methods of tests of burnt clay building bricks; Determination of compressive strength, Bureau of Indian Standards, (1992).
IS-1077 Common Burnt Clay Building Bricks – Specification. Bureau of Indian Standards, (2007).
IS 3495: Part 1 Methods of tests of burnt clay building bricks; Determination of compressive strength, Bureau of Indian Standards, (1992).
British Standards Institution, Specification for the Assessment of the Life Cycle Greenhouse Gas Emissions of Goods and Services (2011).
Cefic, E. C. T. A. Guidelines for measuring and managing CO2 emission from freight transport operations. Cefic. Rep. 1(2011), 1–18 (2011).
A. C. McKinnon A.C., Piecyk, M., Measuring and managing CO2 emissions in European chemical transport (2010).
Kulkarni, N. G. & Rao, A. B. Carbon footprint of solid clay bricks fired in clamps of India. J. Clean. Prod. 135, 1396–1406 (2016).
Bureau of Indian Standard, IS 2720 (Part XXIII: 1976): Methods of Tests for Soils: Determination of calcium carbonate (2006).
Deo, A., Fuel consumption from light commercial vehicles in India, fiscal year 2018–19. International Council on Clean Transportation (2021).
Kishore, E. A., Carbon emissions from Indian Railways (2012).
https://www.bts.gov/content/energy-consumption-mode-transportation.
G. Hammond, C. Jones, Inventory of carbon and energy; Embodied carbon: A BSRIA guide. (2022)
IS 3792 Guide for heat insulation of non-industrial buildings, Bureau of Indian Standards, (1978).
Singh, B., Rahman, M. R., Paswan, R. & Bhattacharyya, S. K. Effect of activator concentration on the strength, ITZ and drying shrinkage of fly ash/slag geopolymer concrete. Construct. Build. Mater. 118, 171–179 (2016).
Ishwarya, G. A., Singh, B., Deshwal, S. & Bhattacharyya, S. K. Effect of sodium carbonate/sodium silicate activator on the rheology, geopolymerization and strength of fly ash/slag geopolymer pastes. Cement Concrete Compos. 97, 226–238 (2019).
Poinot, T. et al. Beneficial use of boiler ash in alkali-activated bricks. Resour. Conservat. Recycling 128, 1–10 (2018).
Rickard, W. D., Williams, R., Temuujin, J. & Van Riessen, A. Assessing the suitability of three Australian fly ashes as an aluminosilicate source for geopolymers in high temperature applications. Mater. Sci. Eng.: A 528, 3390–3397 (2011).
Schipper, L., Leather, J., & Fabian, H. Transport and carbon dioxide emissions: forecasts, options analysis, and evaluation (2009).
Fernando, S. et al. Environmental evaluation and economic analysis of fly ash-rice husk ash blended alkali-activated bricks. Environ. Impact Assessment Rev. 95, 106784 (2022).
Asan, H. Numerical computation of time lags and decrement factors for different building materials. Build. Environ. 41, 615–620 (2006).
Davies, M. G. The thermal response of an enclosure to periodic excitation: The CIBSE approach. Build. Environ. 29, 217–235 (1994).
Marletta, L., Evola, G., Giuga, M., & Sicurella, F. The admittance method for calculating the internal temperature swing in free running buildings. BSA 2013, (Bozen-Bolzano, Italy: IBPSA-ITALY, 59–68 (2013).
The authors would like to thank Director CSIR-CBRI Roorkee, for motivation and support towards this research work. The authors would also like to thank Dr. S. R. Karade for providing the compressive strength characterisation. Authors are also grateful to Dr. A Aravind Kumar, Dr. Nagesh B. Balam, Mr. Dinesh Kuamr and Mr. Rakesh Solanki for extended support towards this research. This research work is financially supported by in house R&D project (OLP-2204) of CSIR-CBRI, Roorkee, India.
Construction, Automation and Robotics Group, CSIR- Central Building Research Institute, Roorkee, 247667, India
Siddharth Singh, Ravindra Singh Bisht, Soraj Kumar Panigrahi & Sameer Yadav
Building Materials and Environmental Sustainability Group, CSIR- Central Building Research Institute, Roorkee, 247667, India
You can also search for this author in PubMed Google Scholar
You can also search for this author in PubMed Google Scholar
You can also search for this author in PubMed Google Scholar
You can also search for this author in PubMed Google Scholar
You can also search for this author in PubMed Google Scholar
S.S: Conceptualization, Methodology, Data Analysis, Writing- original draft, review and editing. S.M: LCA analysis, manuscript review. R.S.B: Brick machine set-up for brick casting, manuscript review and editing. S.K.P: Supervision, manuscript review, and editing. S.Y: Mix design preparation, brick casting.
The authors declare no competing interests.
Springer Nature remains neutral with regard to jurisdictional claims in published maps and institutional affiliations.
Open Access This article is licensed under a Creative Commons Attribution 4.0 International License, which permits use, sharing, adaptation, distribution and reproduction in any medium or format, as long as you give appropriate credit to the original author(s) and the source, provide a link to the Creative Commons licence, and indicate if changes were made. The images or other third party material in this article are included in the article's Creative Commons licence, unless indicated otherwise in a credit line to the material. If material is not included in the article's Creative Commons licence and your intended use is not permitted by statutory regulation or exceeds the permitted use, you will need to obtain permission directly from the copyright holder. To view a copy of this licence, visit http://creativecommons.org/licenses/by/4.0/.
Singh, S., Maiti, S., Bisht, R.S. et al. Large CO2 reduction and enhanced thermal performance of agro-forestry, construction and demolition waste based fly ash bricks for sustainable construction. Sci Rep 14, 8368 (2024). https://doi.org/10.1038/s41598-024-59012-8
DOI: https://doi.org/10.1038/s41598-024-59012-8
Anyone you share the following link with will be able to read this content:
Sorry, a shareable link is not currently available for this article.
Provided by the Springer Nature SharedIt content-sharing initiative
By submitting a comment you agree to abide by our Terms and Community Guidelines. If you find something abusive or that does not comply with our terms or guidelines please flag it as inappropriate.
Scientific Reports (Sci Rep) ISSN 2045-2322 (online)
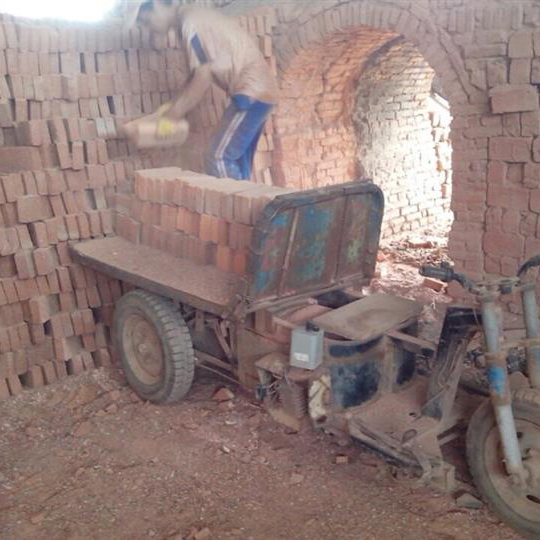
Mixing Machine Sign up for the Nature Briefing newsletter — what matters in science, free to your inbox daily.