Thank you for visiting nature.com. You are using a browser version with limited support for CSS. To obtain the best experience, we recommend you use a more up to date browser (or turn off compatibility mode in Internet Explorer). In the meantime, to ensure continued support, we are displaying the site without styles and JavaScript.
Nature Communications volume 9, Article number: 1754 (2018 ) Cite this article CAS NO 1871-57-4
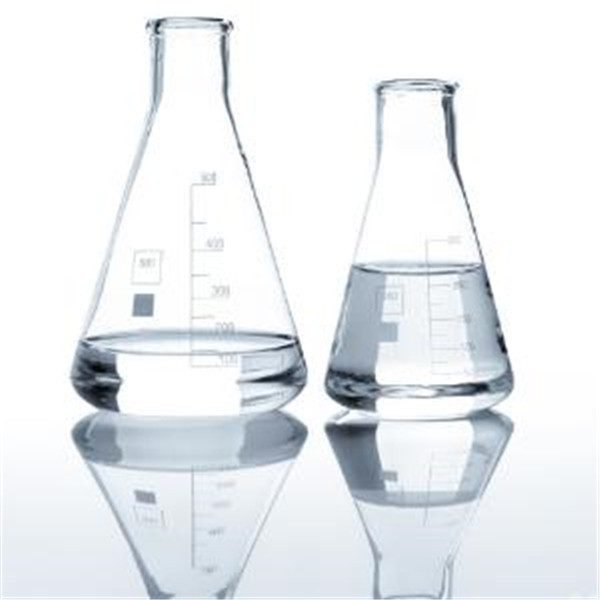
Organocatalytic polymerization reactions have a number of advantages over their metal-catalyzed counterparts, including environmental friendliness, ease of catalyst synthesis and storage, and alternative reaction pathways. Here we introduce an organocatalytic polymerization method called benzylic chloromethyl-coupling polymerization (BCCP). BCCP is catalyzed by organocatalysts not previously employed in polymerization processes (sulfenate anions), which are generated from bench-stable sulfoxide precatalysts. The sulfenate anion promotes an umpolung polycondensation via step-growth propagation cycles involving sulfoxide intermediates. BCCP represents an example of an organocatalyst that links monomers by C=C double bond formation and offers transition metal-free access to a wide variety of polymers that cannot be synthesized by traditional precursor routes.
Simon Edelmann & Jean-Philip Lumb
Hau Sun Sam Chan, Yilin Lu & Jin-Quan Yu
Wanlan Su, Jide Zhu, … Qiuling Song
Innovations in polymer chemistry and materials science often have their genesis in the introduction of small molecule catalysts1,2. This is particularly true in the developing field of organocatalytic polymerization chemistry.3,4 Organocatalytic polymerization reactions have a number of advantages over their metal-catalyzed counterparts, including environmental friendliness, reduced toxicity and cost, ease of catalyst synthesis and storage, and access to alternative reaction pathways. Furthermore, organocatalysts circumvent problems caused by metal residue contamination of polymers, which can severely limit biomedical and electronic applications, and complicate polymer purification and processing3,4. The majority of organocatalytic polymerizations involve ring-opening polymerizations using cyclic esters, carbonates, ethers, siloxanes, anhydrides, and phosphoesters4.
Herein we introduce a class of organocatalytic polymerization processes termed benzylic chloromethyl-coupling polymerization (BCCP). BCCP represents the application of sulfenate anion organocatalysts to polymerization processes. The sulfenate anion-catalyzed process proceeds via an umpolung mechanism and represents a rare example of an organocatalysts that enchains monomers by C=C bond formation5,6. Design of BCCP is validated in the context of poly(m-phenylene vinylene) (PmPV) synthesis. In this study, PmPV’s with Mn as high as 17,400 Da and with very high trans-selectivity are obtained. To demonstrate the mechanistic distinctness of BCCP, a non-conjugated polymer bearing quaternary –C(CF3)2 spacers between stilbene units in the polymer backbone is synthesized. Moreover, two alternating co-polymers as representatives of poly[(1,3-phenylene vinylene)-alt-arene]s and poly[(1,3-phenylene vinylene)-alt-(1,4-phenylene vinylene)] (PmPVpPV)s are synthesized. Nuclear magnetic resonance (NMR) spectra, thermal, photophysical, electrochemical, and charge transport properties of the above mentioned co-polymers are characterized. The polymers reported herein cannot be prepared by classic precursor routes (Gilch, Wesling, and Vanderzande methods).
Sulfenate anions (ArSO–) are highly reactive intermediates in biological chemistry and in organic reactions7,8,9,10. We recently disclosed that sulfenate anions can act as organocatalysts and reported their ability to catalytically dehydrocouple benzyl halides under basic conditions to yield trans-stilbenes (Fig. 1a)11 and their application to catalytic cross-coupling of benzyl chlorides with benzaldehyde derivatives to produce diarylacetylenes12. The efficiency of sulfenate anion catalysts in these reactions, and their high selectivity for formation of trans-stilbenes, inspired us to explore their potential in polymerization reactions. We hypothesized that substrates bearing two benzylic chloromethyl groups would be suitable monomers for polymerization. The benzylic chloromethyl substituents could be located on the same aromatic system or on different aromatic rings separated by linking groups, as represented in Fig. 1b.
Sulfenate anion-catalyzed reactions. a Dehydrocoupling of benzyl chlorides to produce stilbenes. b Generic representation of the benzylic chloromethyl-coupling polymerization (BCCP), where the blue box represents an aromatic system or tethered aromatic rings. c Fundamental steps in the organocatalytic BCCP
Based on this hypothesis, we designed the 1,3-bis(chloromethyl)benzene monomer A (Fig. 1c). We envisioned that the sulfenate anion would react with monomer A via an SN2 reaction to generate sulfoxide B. In the presence of base, sulfoxide B is reversibly deprotonated to generate carbanion C. Anion C is a reactive nucleophile and undergoes SN2 with monomer A to form the first C–C bond. Base promoted E2 elimination of intermediate D provides dimer E and liberates the sulfenate anion to further catalyze the polycondensation of E. Notably, the product is a PmPV, which is an important class of organic semiconductors with applications in optoelectronics, such as organic light-emitting diodes (OLEDs), solar cells, organic lasers, sensors, and displays13,14,15,16,17,18. Although the synthesis of PPV’s has been developed, including precursor routes19,20,21,22,23, olefin metathesis polymerizations23, nucleophilic condensations24,25,26,27,28,29, and cross-coupling polymerizations30,31,32,33,34,35,36, to the best of our knowledge this is a unique organocatalytic method for the synthesis of this important class of polymers. Moreover, PmPV is a challenging target, because meta-linkages preclude formation of quinodimethane intermediates, prohibiting classic PPV precursor routes (Gilch, Wesling and Vanderzande methods)33,37,38,39,40.
Starting from the optimized coupling of benzyl chlorides used in our stilbene synthesis (Fig. 1a)11, we selected cyclopentyl methyl ether (CPME) as solvent and KOtBu as base at 80 oC, to optimize the polymerization of monomer M1 (Fig. 2). A long alkyl chain was introduced onto the PmPV backbone to assure the resulting polymer P1 has good solubility in common organic solvents. Initial reactions were conducted in 24-well plates on 10 µmol scale by adapting small molecule high-throughput experimentation (HTE)41,42,43,44,45,46,47,48 techniques to polymerizations (see Supplementary Method, High-Throughput Experimentation screenings for polymerization for full details). As shown in Fig. 2a, we initially focused on air-stable benzylic sulfoxide catalysts (1–9) ArSOCH2Ph with various Ar–S groups and one precatalyst (10) with 4 catalyst loadings (10, 7.5, 5.0, and 2.5 mol %). Reactions were heated for 24 h at 80 oC followed by cooling and work up by addition of 10 µL of water and removal of the volatile materials. Next, CHCl3 was added to each well to dissolve the products followed by cold methanol to precipitate the solid polymer. Finally, filtration of the solid, dissolution in tetrahydrofuran (THF) and analysis by gel permeation chromatography (GPC) against polystyrene standards were performed. In this screen we observed complete polymerization at 10, 7.5, and 5.0 mol % catalyst loadings. There was little impact of the substituents on the aryl ring of the sulfenate anion (ArSO–), with similar Mn and polydispersity index (PDI) (Mn ~ 10,000 were observed at 10 and 7.5 mol % loading and Mn ~ 9000 at 5.0 mol % loading). Lower catalyst loadings of 2.5 mol % led to oligomerization (see Supplementary Table 1). Moreover, we observed complete consumption of monomer M1 after 10 min. A rapid loss of monomer at the beginning of the polymerization indicates that the BCCP proceeds by a step-growth mechanism.
Optimization by HTE Screening. a Precatalysts employed leading to sulfoxide 10 for further studies. b Additional base screen indicated that NaN(SiMe3)2 (Mn = 10,200) gave the best results, but lower than KOtBu (Mn = 10,900) of panel a. c Additional solvent screen, wherein MTBE gave the best results (Mn = 13,200) higher than CPME of panel a. See Supplementary Table 3 for all results
At this stage of our investigations we chose to employ precatalyst 10. Under the basic conditions of the polymerization, 10 rapidly undergoes E2 elimination to form styrene and generate the sulfenate anion12. The most promising results with precatalyst 10 were with 7.5 mol % (Mn 10,900, PDI 1.26). At this loading, we conducted a second screen focused on 10 bases [LiOtBu, NaOtBu, LiN(SiMe3)2, NaN(SiMe3)2, KN(SiMe3)2, NaH, KH, KOSiMe3, KOPh, NaOMe] under otherwise identical conditions (Fig. 2b). Analysis of the resulting reactions indicated that polymer was obtained only with LiN(SiMe3)2, NaN(SiMe3)2, KN(SiMe3)2 with Mn all lower than with KOtBu from the first screen (see Supplementary Table 2).
The next step in the optimization was a solvent screen. We examined five solvents (THF, dioxane, MTBE (methyl tert-butyl ether), toluene, and dimethylformamide). As shown in Fig. 2C, the most promising result was obtained in MTBE (Mn 13,200, PDI 1.23).
After narrowing our optimization parameters to precatalyst 10 (7.5 mol %), KOtBu, and CPME and MTBE as two top solvents, we conducted lab-scale (0.1 mmol) polymerizations to validate the microscale results and further optimize the BCCP (Table 1). Lab-scale polycondensation of monomer M1 with both CPME and MTBE at 0.05 M concentration yielded polymer with Mn 10,400, PDI 1.28 in 91% isolated yield for CPME and polymer with Mn 13,600, PDI 1.21 in 69% isolated yield with MTBE. The results confirmed that Mn and PDI of polymers obtained at 10 µmol scale could be reproduced at 0.1 mmol scale. With CPME as solvent, increasing concentration to 0.1 M and 0.2 M (entries 3–4) led to higher Mn (11,200 at 0.1 M and 12,200 at 0.2 M) with similar PDIs. The yields, however, dropped from 91% (0.05 M) to 86% (0.1 M) and 73% (0.2 M). With MTBE as solvent, increasing concentration led to higher Mn of 15,300 (0.1 M, entry 5) in 72% yield and 17,400 (0.2 M, entry 6) in 71% yield, with PDI of the corresponding polymers of 1.41. Employing 4 and 5 equivalents of KOtBu afforded polymer product with similar Mn, PDI, and yield (entries 7–8). The Mn dropped to 10,200 when 6 equiv. of base were employed (entry 9).
Scalability is an important attribute of polymerization catalysts. We next scaled the BCCP of M1 to 1 mmol scale using the conditions outlined in Table 1, entry 1. Under the reaction conditions shown in Fig. 3, the polymer P1 was obtained with Mn 10,600 and PDI 1.20 in 90% yield (334.8 mg).
Scale-up of P1. Synthesis of P1 by BCCP reaction
Traditionally, PPVs were synthesized by a two-step quinodimethane polymerization/elimination protocol (the precursor route)19. The quinodimethane polymerization forms a non-conjugated polymer that is then converted to the conjugated PPV by high-temperature (180–300 oC) thermal elimination reaction (Fig. 4a)49. The harsh conditions required for converting non-conjugated precursor polymers to PPVs and the incomplete elimination lead to structural defects, which affect the luminescence quantum efficiency of the PPV films50. In sharp contrast, sulfenate anion-catalyzed BCCP proceeds by a different mechanism, which results in chemoselective construction of trans double bonds (Fig. 4b). Compared with other common methods for the preparation of PPVs such as transition metal-mediated Heck reactions, cross-coupling reactions, and metathesis reactions51, BCCP is a transition metal-free process.
Comparison of BCCP polymerization with precursor routes. a Summary of precursor route. b BCCP polymerization
To highlight the advantage of BCCP over precursor routes (Gilch, Wesling, and Vanderzande methods) (Fig. 4a), we designed monomer M2 in which two benzyl chloromethyl groups are linked by a C(CF3)2 bridge (Fig. 5). As the C(CF3)2 linker prevents the formation of quinodimethane intermediate, polymer P2 could not be prepared by precursor routes (Fig. 4a). Using the conditions in Table 1 (entry 1), the BCCP afforded polymer P2 in 82% yield with Mn 14,300 and PDI 1.45.
Synthesis of polymer P2. The polymer generated has a C(CF3)2 linker
It is well-known that OLED device performance is greatly influenced by the structural regularity of the polymers. To further demonstrate the synthetic potential of BCCP, we next employed BCCP in the synthesis of challenging alternating copolymers. We designed a class of monomers (M3, Fig. 6a) by incorporating a flourenyl group between two meta-phenyl groups. Polymerization of monomer M3 bearing different central Ar groups is expected to lead to a new class of structurally regular and alternating poly[(1,3-phenylene vinylene)-alt-arene]s. As proof-of-concept, we synthesized a fluorine-containing monomer M3-1. BCCP of M3-1 led to co-polymer P3-1 in 91% yield with Mn 13,000 and PDI 2.00 (see Supplementary Method, 1 mmol Scale synthesis and characterization of co-polymer P3-1, P4-1, for the synthesis of monomer M3-1 and co-polymer P4-1).
Design and synthesis of alternating co-polymers. a Poly[(1,3-phenylene vinylene)-alt-arene]s. b Poly[(1,3-phenylene vinylene)-alt-(1,4-phenylene vinylene)] (PmPVpPV)s
Previous studies have shown that (PmPVpPV)s are highly photoluminescent polymers with well-defined conjugation lengths52,53,54,55. Such observations inspired us to design a member of this class, monomer M4. We expect that polymerization of M4 type monomers will achieve the synthesis of structurally regular (PmPVpPV)s with 1:1 alternating mPV and pPV units along the chain linked by trans C=C bonds (Fig. 6b). Moreover, incorporation of different moieties as side-chains into the PmPVpPV backbone (R group on the pPV unit of P4), would allow tuning of the emission wavelength, emission color and change the quantum efficiency of the resulting co-polymer P4. As a proof-of-concept, we synthesized a thiophene-containing monomer M4-1. BCCP of M4-1 led to co-polymer P4-1 in 93% yield with Mn 8000 and PDI 1.82 (see Supplementary Method, 1 mmol Scale synthesis and characterization of co-polymer P3-1, P4-1, for the synthesis of monomer M4-1 and co-polymer P4-1). Notably, both polymers P3-1 and P4-1 are not accessible by the precursor route, demonstrating the value of BCCP in co-polymer synthesis.
The thermal, photophysical, electrochemical and charge transport properties of P3-1 and P4-1 were characterized (see Supplementary Figure 37). As shown in Fig. 7a, I, the decomposition temperatures (Td, corresponding to 5% weight loss) measured from thermogravimetric analysis were 420 oC and 404 oC for P3-1 and P4-1, respectively, indicating good thermal stability. Thermal stability is valuable for long device operation in emission materials15. From the glass transition temperatures (Tg) observed from differential scanning calorimetry (DSC) for the more rigid fluorene containing P3-1 was 141 oC; however, no obvious phase transition temperature could be obtained for P4-1 with more flexible C–C double bonds in the conjugated backbone (Fig. 7b). Both polymers showed bright emission under UV excitation, as shown in the photo images of Fig. 7c,d, II. From the UV-Vis spectra in both solution and film (Fig. 7c,d), it is found that two-dimensional conjugated P4-1 with the pendant thiophene ring exhibited broader and red-shifted absorption relative to P3-1, with a one-dimensional conjugated polymer backbone. Regarding the photoluminescence (PL), the thiophene-containing P4-1 displayed red-shifted PL compared with P3-1, with emission peaks in the deep blue (435 nm in THF and 457 nm in the film state) for P4-1 and ultra-violet (373 nm in THF and 402 nm in the film state) for P3-1. The electrochemical properties were measured by cyclic voltammetry (Fig. 7e). Both polymers exhibited quasi-reversible oxidation and irreversible reduction behavior. The highest occupied molecular orbital (HOMO) and lowest unoccupied molecular orbital (LUMO) energy levels were determined from the onset of oxidation and reduction curves for P3-1 and P4-1, and were calculated to be −5.97/−2.60 eV and −5.65/−2.77 eV, respectively. Compared with P3-1, the introduction of a strong electron-donating thiophene ring in P4-1 significantly raises the HOMO level by 0.32 eV, suggesting a more efficient hole-injection and better hole transport properties of P4-1 in optoelectronic devices. This prediction is in good agreement with the measured hole mobility from the space charge limited current method (Fig. 7f). The hole mobility for the thiophene-containing P4-1 is estimated to be 1.56 × 10−6 cm2 V−1 s−1, which doubles P3-1 of 7.79 × 10−7 cm2 V−1 s−1.
Polymer characterization. a TGA curves of the co-polymers P3-1 and P4-1. b DSC curves of the co-polymers P3-1 and P4-1. c Normalized UV-Vis absorption and PL spectra of co-polymers P3-1 and P4-1 in THF solution and photographs of polymer photoluminescence under 365 nm light in THF solution. d Normalized UV-Vis absorption and PL spectra of co-polymers P3-1 and P4-1 in film state and photographs of polymer photoluminescence under 365 nm light in solid state. e Cyclic voltammograms of the oxidation curves in dichloromethane and reduction curves in THF for co-polymers P3-1 and P4-1. f Current density–voltage (J–V) characteristics of hole-only devices with structure of ITO/PEDOT:PSS(30 nm)/P3-1 or P4-1 (30 nm)/MoO3 (8 nm)/Ag
Introduced herein is a class of organocatalytic polymerization processes termed BCCP. The organocatalysts for this process, sulfenate anions, are operationally trivial to generate from bench-stable sulfoxide precatalysts in the presence of base. Sulfenate anion organocatalysts are unique in that they enable generation of C=C double bonds of the type found in PPV’s and other stilbene-based polymers. We demonstrated the application of sulfenate anion-catalyzed transfer polycondensation methods to polymers bearing isolated stilbene motifs. The important conceptual advance of this work is that it suggests that small organic molecules that can activate substrates via nucleophilic attack, acidify neighboring hydrogens leading to umpolung reactivity, and then behave as leaving groups can be considered in polymerization processes to forge C=C linkages. From the synthetic aspect, BCCP offers transition metal-free access to wide varieties of polymers that cannot be synthesized by traditional precursor routes (Gilch, Wesling, and Vanderzande methods). To further demonstrate the synthetic potential of BCCP, two alternating co-polymers were synthesized as representatives of classes of poly[(1,3-phenylene vinylene)-alt-arene]s and (PmPVpPV)s. NMR spectra, thermal, photophysical, electrochemical, and charge transport properties of the above mentioned polymers were characterized. Further studies are underway to apply BCCP to the preparation of novel functionalized polymers.
An oven-dried 8 mL microwave vial equipped with a stir bar was charged with monomer M1 (44.4 mg, 0.10 mmol) under a nitrogen atmosphere in a glove box. A solution of precatalyst 10 (1.73 mg, 0.0075 mmol) in 1.0 mL anhydrous CPME was added by syringe. Next, a solution of KOtBu (33.6 mg, 0.30 mmol) in 1.0 mL anhydrous CPME was added by syringe. The reaction was stirred for 24 h at 80 °C, quenched with 2 drops of H2O via syringe, cooled to room temperature, and opened to air. After the volatile materials were removed with a rotary evaporator, CHCl3 (2 mL) was added into each vial and the slurry solution was allowed to stir for 10 min. Cold methanol (6 mL) and H2O (0.5 mL) was then added into each vial to precipitate the polymer and the slurry solution with polymer suspension was allowed to stir for 10 min. The mixture was then transferred with a pipette onto a Whatman autovial syringeless filter (5 mL, 0.45 µm polytetrafluoroethylene (PTFE) membrane). After the MeOH/CHCl3/H2O solution was filtered, polymer that remained in the filter was washed sequentially with 5 mL MeOH and 5 mL pentane. Finally, the polymer remaining in the filter was transferred into a 20 mL vial with spatula and dried under vacuum to yield a pale yellow solid in 33.8 mg, 91% yield.
An oven-dried 100 mL Schlenk tube equipped with a stir bar was charged with monomer 1 (444.0 mg, 1.0 mmol) and precatalyst 10 (17.3 mg, 0.075 mmol). The Schlenk tube was sealed with a rubber septum and was connected to a Schlenk line, evacuated, and refilled with nitrogen (repeated three times). Next, a solution of KOtBu (336 mg, 3.0 mmol) in 20 mL anhydrous CPME was added by syringe. The reaction was stirred for 24 h at 80 °C, cooled to room temperature, opened to air, and quenched with 1 mL of H2O. The reaction mixture was firstly transferred to a 250 mL round-bottom flask and the volatile materials were removed with a rotary evaporator. Next, CHCl3 (20 mL) was added into flask and the slurry solution was allowed to stir for 10 min. Cold methanol (60 mL) was added into the flask to precipitate the polymer and the slurry solution with polymer suspension was allowed to stir for 10 min. The mixture was then filtered on a glass fritted filter funnel (75 mL). After the MeOH/CHCl3 solution was filtered, the resulting solid was washed with H2O (5 mL), MeOH (20 mL *3), and pentane (5 mL). The solid was collected and dried in a vacuum as pale yellow solid to provide 334.8 mg, 90% yield of the polymer.
The authors declare that the data supporting the findings of this study are available within the article and its Supplementary Information files.
Ober, C. K. et al. Research in macromolecular science: challenges and opportunities for the next decade. Macromolecules 42, 465–471 (2009).
Article ADS CAS Google Scholar
Hawker, C. J. & Wooley, K. L. The convergence of synthetic organic and polymer chemistries. Science 309, 1200–1205 (2005).
Article ADS CAS PubMed Google Scholar
Kiesewetter, M. K., Shin, E. J., Hedrick, J. L. & Waymouth, R. M. Organocatalysis: opportunities and challenges for polymer synthesis. Macromolecules 43, 2093–2107 (2010).
Article ADS CAS Google Scholar
Ottou, W. N., Sardon, H., Mecerreyes, D., Vignolle, J. & Taton, D. Update and challenges in organo-mediated polymerization reactions. Prog. Polym. Sci. 56, 64–115 (2016).
Hong, M. & Chen, E. Y. Proton-transfer polymerization (HTP): converting methacrylates to polyesters by an N-heterocyclic carbene. Angew. Chem. 53, 11900–11906 (2014).
Hong, M. et al. Proton-transfer polymerization by N-heterocyclic carbenes: monomer and catalyst scopes and mechanism for converting dimethacrylates into unsaturated polyesters. J. Am. Chem. Soc. 138, 2021–2035 (2016).
Article CAS PubMed Google Scholar
Maitro, G. et al. Enantioselective synthesis of aryl sulfoxides via palladium-catalyzed arylation of sulfenate anions. Org. Lett. 9, 5493–5496 (2007).
Article CAS PubMed Google Scholar
O’Donnell, J. S. & Schwan, A. L. Generation, structure and reactions of sulfenic acid anions. J. Sulfur Chem. 25, 183–211 (2004).
Schwan, A. L. & Söderman, S. C. Discoveries in sulfenic acid anion chemistry. Phosphorus Sulfur. Silicon Relat. Elem. 188, 275–286 (2013).
Soderman, S. C. & Schwan, A. L. Sulfenate substitution as a complement and alternative to sulfoxidation in the diastereoselective preparation of chiral beta-substituted beta-amino sulfoxides. J. Org. Chem. 78, 1638–1649 (2013).
Article CAS PubMed Google Scholar
Zhang, M. et al. A new class of organocatalysts: sulfenate anions. Angew. Chem. Int. Ed. Engl. 53, 10755–10758 (2014).
Article CAS PubMed Google Scholar
Zhang, M., Jia, T., Wang, C. Y. & Walsh, P. J. Organocatalytic synthesis of alkynes. J. Am. Chem. Soc. 137, 10346–10350 (2015).
Article CAS PubMed Google Scholar
Wang, S. et al. A DRIFTS study of low-temperature CO oxidation over Au/SnO2 catalyst prepared by co-precipitation method. Catal. Commun. 10, 640–644 (2009).
Cheng, Y. J., Yang, S. H. & Hsu, C. S. Synthesis of conjugated polymers for organic solar cell applications. Chem. Rev. 109, 5868–5923 (2009).
Article CAS PubMed Google Scholar
Gunes, S., Neugebauer, H. & Sariciftci, N. S. Conjugated polymer-based organic solar cells. Chem. Rev. 107, 1324–1338 (2007).
Wang, S. et al. Porous ceria hollow microspheres: synthesis and characterization. Microporous Mesoporous Mater. 123, 349–353 (2009).
Spanggaard, H. & Krebs, F. C. A brief history of the development of organic and polymeric photovoltaics. Sol. Energ. Mat. Sol. Cells 83, 125–146 (2004).
Grimsdale, A. C., Chan, K. L., Martin, R. E., Jokisz, P. G. & Holmes, A. B. Synthesis of light-emitting conjugated polymers for applications in electroluminescent devices. Chem. Rev. 109, 897–1091 (2009).
Article CAS PubMed Google Scholar
Junkers, T., Vandenbergh, J., Adriaensens, P., Lutsen, L. & Vanderzande, D. Synthesis of poly(p-phenylene vinylene) materials via the precursor routes. Polym. Chem. 3, 275–285 (2012).
Becker, H., Spreitzer, H., Ibrom, K. & Kreuder, W. New insights into the microstructure of GILCH-polymerized PPVs. Macromolecules 32, 4925–4932 (1999).
Article ADS CAS Google Scholar
Gilch, H. G. & Wheelwright, W. L. Polymerization of α-halogenated p-xylenes with base. J. Polym. Sci. A Polym. Chem. 4, 1337–1349 (1966).
Article ADS CAS Google Scholar
Ravnsbæk, J. B. & Swager, T. M. Mechanochemical synthesis of poly(phenylene vinylenes). ACS Macro. Lett. 3, 305–309 (2014).
Zaquen, N., Lutsen, L., Vanderzande, D. & Junkers, T. Controlled/living polymerization towards functional poly(p-phenylene vinylene)materials. Polym. Chem. 7, 1355–1367 (2016).
McCullough, R. D. & Lowe, R. D. Enhanced electrical conductivity in regioselectively synthesized poly(3-alkylthiophenes). J. Chem. Soc. Chem. Commun. 0, 70–72 (1992).
Lenz, R. W. & Handlovits, C. E. Thermally stable hydrocarbon polymers: polyterephthalylidenes. J. Org. Chem. 25, 813–817 (1960).
Suzuki, Y., Hashimoto, K. & Tajima, K. Synthesis of regioregular poly(p-phenylenevinylene)s by Horner reaction and their regioregularity characterization. Macromolecules 40, 6521–6528 (2007).
Article ADS CAS Google Scholar
Schenk, R., Gregorius, H., Meerholz, K., Heinze, J. & Muellen, K. Novel oligo(phenylenevinylenes): models for the charging of extended.pi. chains. J. Am. Chem. Soc. 113, 2634–2647 (1991).
Yang, Z. & Geise, H. J. Preparation and electrical conductivity of blends consisting of modified Wittig poly(para-phenylene vinylene), iodine and polystyrene, polymethyl methacrylate or polycarbonate. Synt. Met. 47, 105–109 (1992).
Conticello, V. P., Gin, D. L. & Grubbs, R. H. Ring-opening metathesis polymerization of substituted bicyclo[2.2.2]octadienes: a new precursor route to poly(1,4-phenylenevinylene). J. Am. Chem. Soc. 114, 9708–9710 (1992).
Zou, Y., Hou, J., Yang, C. & Li, Y. A novel n-type conjugated polymer DOCN-PPV: synthesis, optical, and Eeectrochemical properties. Macromolecules 39, 8889–8891 (2006).
Article ADS CAS Google Scholar
Schneider, J. A., Dadvand, A., Wen, W. & Perepichka, D. F. Tuning the electronic properties of poly(thienothiophene vinylene)s via alkylsulfanyl and alkylsulfonyl substituents. Macromolecules 46, 9231–9239 (2013).
Article ADS CAS Google Scholar
Bao, Z., Chan, W. K. & Yu, L. Exploration of the Stille coupling reaction for the synthesis of functional polymers. J. Am. Chem. Soc. 117, 12426–12435 (1995).
Wakioka, M., Ikegami, M. & Ozawa, F. Stereocontrolled synthesis and photoisomerization behavior of all-cis and all-trans poly(m-phenylenevinylene)s. Macromolecules 43, 6980–6985 (2010).
Article ADS CAS Google Scholar
Katayama, H. et al. Stereocontrolled synthesis and characterization of cis-poly(arylenevinylene)s. Macromolecules 39, 2039–2048 (2006).
Article ADS CAS Google Scholar
Katayama, H. et al. Stereocontrolled synthesis and optical properties of all-cis poly(phenylene vinylenes) (PPVs): a method for direct patterning of PPVs. J. Am. Chem. Soc. 127, 4350–4353 (2005).
Article CAS PubMed Google Scholar
Yu, L., Lee, Y. & Liang, Y. The Heck polycondensation for functional polymers. Synlett 2006, 2879–2893 (2006).
Cyriac, A., Amrutha, S. R. & Jayakannan, M. Renewable resource-based poly (m-phenylenevinylene)s and their statistical copolymers: synthesis, characterization, and probing of molecular aggregation and Forster energy transfer processes. J. Polym. Sci. A Polym. Chem. 46, 3241–3256 (2008).
Article ADS CAS Google Scholar
Drury, A. et al. Systematic trends in the synthesis of (meta-phenylene vinylene) copolymers. Synth. Met. 119, 151–152 (2001).
Liao , L. , Pang , Y. , Ding , L. & Karasz , FE Blue-emitting soluble poly(m-phenylenevinylene) derivatives.Macromolecules 34, 7300–7305 (2001).
Article ADS CAS Google Scholar
Liao, L., Pang, Y., Ding, L. & Karasz, F. E. Synthesis, characterization and luminescence of poly[(m-phenylenevinylene)-alt-(1,4-dibutoxy-2,5-phenylenevinylene)] with different content of cis- and trans-Olefins. Macromolecules 34, 6756–6760 (2001).
Article ADS CAS Google Scholar
Zhang, H., Marin, V., Fijten, M. W. M. & Schubert, U. S. High-throughput experimentation in atom transfer radical polymerization: a general approach toward a directed design and understanding of optimal catalytic systems. J. Polym. Sci. A Polym. Chem. 42, 1876–1885 (2004).
Article ADS CAS Google Scholar
Potyrailo, R. et al. Combinatorial and high-throughput screening of materials libraries: review of state of the art. ACS Comb. Sci. 13, 579–633 (2011).
Article CAS PubMed Google Scholar
Li, M. et al. Transition-metal-free radical C(sp(3))-C(sp(2)) and C(sp(3))-C(sp(3)) coupling enabled by 2-Azaallyls as super-electron-donors and coupling-partners. J. Am. Chem. Soc. 139, 16327–16333 (2017). For High-throughput screening in small molecule synthesis.
Article CAS PubMed Google Scholar
Li, M., Berritt, S. & Walsh, P. J. Palladium-catalyzed regioselective arylation of 1,1,3-triaryl-2-azaallyl anions with aryl chlorides. Org. Lett. 16, 4312–4315 (2014).
Article CAS PubMed PubMed Central Google Scholar
Li, M. et al. Palladium-catalyzed C-H arylation of α,β-unsaturated imines: catalyst-controlled synthesis of enamine and allylic amine derivatives. Angew. Chem. Int. Ed. 55, 2825–2829 (2016).
Li, M. et al. Transition-metal-free chemo- and regioselective vinylation of azaallyls. Nat. Chem. 9, 997–1004 (2017).
Article CAS PubMed Google Scholar
Li, M., Yucel, B., Adrio, J., Bellomo, A. & Walsh, P. J. Synthesis of diarylmethylamines via palladium-catalyzed regioselective arylation of 1,1,3-triaryl-2-azaallyl anions. Chem. Sci. 5, 2383–2391 (2014).
Article CAS PubMed PubMed Central Google Scholar
Li, M. et al. Umpolung synthesis of diarylmethylamines via palladium- catalyzed arylation of N-benzyl aldimines. Adv. Synth. Catal. 358, 1910–1915 (2016).
Article ADS CAS PubMed PubMed Central Google Scholar
van Breemen, A. J. J. M. et al. Optimization of the polymerization process of sulfinyl precursor polymers toward poly(p-phenylenevinylene). Macromolecules 32, 5728–5735 (1999). For High-throughput screening in macromolecule synthesis.
Anuragudom, P., Newaz, S. S., Phanichphant, S. & Lee, T. R. Facile Horner−Emmons synthesis of defect-free poly(9,9-dialkylfluorenyl-2,7-vinylene). Macromolecules 39, 3494–3499 (2006).
Article ADS CAS Google Scholar
Babudri, F., Farinola, G. M. & Naso, F. Synthesis of conjugated oligomers and polymers: the organometallic way. J. Mater. Chem. 14, 11–34 (2004).
Liao, L., Cirpan, A., Ding, L., Karasz, F. E. & Pang, Y. Efficient blue-green-emitting poly[(5-diphenylamino-1,3-phenylenevinylene)-alt-(2,5-dihexyloxy-1,4-phenylenevinylene)] derivatives: synthesis and optical properties. J. Polym. Sci. A. 44, 2307–2315 (2006).
Yang, L. J. et al. Host-guest system of hesperetin and beta-cyclodextrin or its derivatives: preparation, characterization, inclusion mode, solubilization and stability. Mater. Sci. Eng. C. Mater. Biol. Appl. 59, 1016–1024 (2016).
Article CAS PubMed Google Scholar
Yang, L.-J. et al. Host–guest interaction between brazilin and hydroxypropyl-β-cyclodextrin: preparation, inclusion mode, molecular modelling and characterization. Dyes Pigm. 150, 193–201 (2018).
Yang, L.-J. et al. Supramolecular system of podophyllotoxin and hydroxypropyl-β-cyclodextrin: characterization, inclusion mode, docking calculation, solubilization, stability and cytotoxic activity. Mater. Sci. Eng. C. 76, 1136–1145 (2017).
P.J.W. thanks the National Science Foundation (CHE-1464744). We thank Professor Jeung Gon Kim of Chonbuk National University and Dr. Jerome Robinson of UPenn for helpful discussions. C.W. thanks the Vagelos Integrated Program in Energy Research for funding and support.
Roy and Diana Vagelos Laboratories, Penn/Merck Laboratory for High-Throughput Experimentation, Department of Chemistry, University of Pennsylvania, 231 South 34th Street, Philadelphia, Pennsylvania, 19104, USA
Minyan Li, Simon Berritt, Carol Wang, Xiaodong Yang, Yang Liu, Sheng-Chun Sha, Bo Wang & Patrick J. Walsh
Key Laboratory of Medicinal Chemistry for Natural Resources, Ministry of Education and Yunnan Province, School of Chemical Science and Technology, Yunnan University, Kunming, 650091, P. R. China
Institute of Advanced Synthesis, School of Chemistry and Molecular Engineering, Jiangsu National Synergetic Innovation Center for Advanced Materials, Nanjing Tech University, 30 South Puzhu Road, Nanjing, 211816, China
Rui Wang, Xinyuan Fan & Patrick J. Walsh
Key Laboratory of Flexible Electronics (KLOFE) and Institute of Advanced Materials (IAM), Nanjing Tech University, 30 South Puzhu Road, Nanjing, 211816, China
Xuyu Gao & Youtian Tao
Key Laboratory of Functional Polymer Materials, Ministry of Education, Institute of Polymer Chemistry, Nankai University, Tianjin, 300071, China
You can also search for this author in PubMed Google Scholar
You can also search for this author in PubMed Google Scholar
You can also search for this author in PubMed Google Scholar
You can also search for this author in PubMed Google Scholar
You can also search for this author in PubMed Google Scholar
You can also search for this author in PubMed Google Scholar
You can also search for this author in PubMed Google Scholar
You can also search for this author in PubMed Google Scholar
You can also search for this author in PubMed Google Scholar
You can also search for this author in PubMed Google Scholar
You can also search for this author in PubMed Google Scholar
You can also search for this author in PubMed Google Scholar
You can also search for this author in PubMed Google Scholar
P.J.W. conceived of the project. M.L and P.J.W. designed the experiments and monomers. M.L., S.B., C.W., X.Y., Y.L., S.-C.S., B.W., X.F., and R.W. performed the research. Z.L. conducted GPC analysis of co-polymers P3-1 and P4-1. X.G. and Y.T. performed the thermal, photophysical, electrochemical, and charge transport properties characterization. M.L. and P.J.W. wrote the paper.
Correspondence to Patrick J. Walsh.
The authors declare no competing interests.
Publisher's note: Springer Nature remains neutral with regard to jurisdictional claims in published maps and institutional affiliations.
Open Access This article is licensed under a Creative Commons Attribution 4.0 International License, which permits use, sharing, adaptation, distribution and reproduction in any medium or format, as long as you give appropriate credit to the original author(s) and the source, provide a link to the Creative Commons license, and indicate if changes were made. The images or other third party material in this article are included in the article’s Creative Commons license, unless indicated otherwise in a credit line to the material. If material is not included in the article’s Creative Commons license and your intended use is not permitted by statutory regulation or exceeds the permitted use, you will need to obtain permission directly from the copyright holder. To view a copy of this license, visit http://creativecommons.org/licenses/by/4.0/.
Li, M., Berritt, S., Wang, C. et al. Sulfenate anions as organocatalysts for benzylic chloromethyl coupling polymerization via C=C bond formation. Nat Commun 9, 1754 (2018). https://doi.org/10.1038/s41467-018-04095-x
DOI: https://doi.org/10.1038/s41467-018-04095-x
Anyone you share the following link with will be able to read this content:
Sorry, a shareable link is not currently available for this article.
Provided by the Springer Nature SharedIt content-sharing initiative
By submitting a comment you agree to abide by our Terms and Community Guidelines. If you find something abusive or that does not comply with our terms or guidelines please flag it as inappropriate.
Nature Communications (Nat Commun) ISSN 2041-1723 (online)
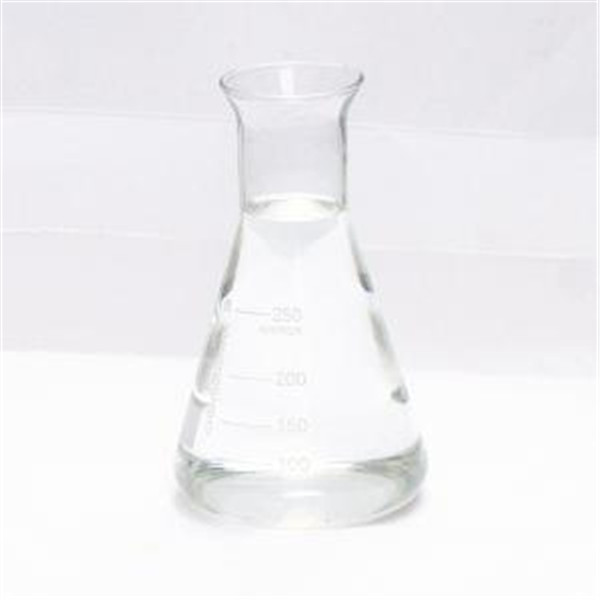
CAS NO 513-42-8 Sign up for the Nature Briefing newsletter — what matters in science, free to your inbox daily.