Thank you for visiting nature.com. You are using a browser version with limited support for CSS. To obtain the best experience, we recommend you use a more up to date browser (or turn off compatibility mode in Internet Explorer). In the meantime, to ensure continued support, we are displaying the site without styles and JavaScript.
Scientific Reports volume 14, Article number: 1594 (2024 ) Cite this article Plywood White Melamine

Lung cancer is a leading cause of cancer-related deaths worldwide. Epidermal growth factor receptor (EGFR) driver mutations are crucial for treatment decisions for patients with non-small cell lung cancer (NSCLC). This study aimed to assess the differences in EGFR mutation detection between two companion diagnostic (CDx) tests—the Oncomine Dx Target Test (ODxTT) and the AmoyDx Pan Lung Cancer PCR Panel—and their impact on treatment applicability. To this end, we used an in-house targeted sequencing dataset of 282 samples from 127 EGFR-mutated NSCLC patients to simulate the concordance between the EGFR variants targeted by the ODxTT and AmoyDx panel, the oncogenicity of the variants, and their therapeutic potential. Of the 216 EGFR mutations identified by the in-house panel, 51% were detectable by both CDx tests, 3% were specific to ODxTT, and 46% were not targeted by either test. Most non-targeted mutations did not have oncogenicity and were located outside exons 18–21. Notably, 95% of the mutations detectable by both tests had potential oncogenicity. Furthermore, among the 96 patients harboring actionable EGFR mutations, 97% had mutations detectable by both CDx tests and 1% by ODxTT, while 2% had mutations not covered by either test. These findings suggest that while both CDx tests are effective in detecting almost all actionable EGFR mutations, ODxTT provides slightly broader coverage. These results emphasize the importance of selecting appropriate CDx tests to inform treatment decisions for EGFR-positive NSCLC patients.
Julie George, Lukas Maas, … Roman K. Thomas
Jaclyn LoPiccolo, Alexander Gusev, … Pasi A. Jänne
Alona Sosinsky, John Ambrose, … Nirupa Murugaesu
Lung cancer is a highly prevalent and refractory disease worldwide, accounting for a high number of cancer-related deaths1. The discovery of driver mutations in the epidermal growth factor receptor (EGFR) gene has revolutionized the treatment landscape for a subset of patients with non-small cell lung cancer (NSCLC)2,3. EGFR mutations occur in approximately 15–20% of NSCLC patients and are detected mainly in exon 19 deletions and the p.L858R mutation in exon 21. These mutations confer sensitivity to EGFR tyrosine kinase inhibitors (TKIs), leading to improved clinical outcomes4,5,6.
EGFR TKIs, including gefitinib, erlotinib, afatinib, and osimertinib, have demonstrated remarkable efficacy in patients harboring sensitizing EGFR mutations7,8,9,10. EGFR TKIs bind to the adenosine triphosphate-binding site within the intracellular domain and inhibit tyrosine kinase activity, leading to perturbation of downstream signaling pathways involved in tumor cell proliferation and survival11. EGFR TKIs have been shown to significantly prolong progression-free survival and improve prognosis compared with conventional chemotherapies.
To facilitate treatment decision-making and prolong patient survival, companion diagnostic (CDx) tests are commonly applied in clinical practice for lung cancer patients12. The identification of actionable mutations in tumors helps clinicians to select appropriate targeted therapies. In Japan, two widely used CDx tests—the Oncomine Dx Target Test (ODxTT) and the AmoyDx Pan Lung Cancer PCR Panel (hereafter AmoyDx panel)—are available for patients with NSCLC13,14,15. ODxTT is based on next-generation sequencing analysis16, while the AmoyDx panel uses multiplex real-time PCR-based assay15. Notably, each CDx test analyzes different sites of EGFR mutations. To date, ODxTT can detect 146 types of EGFR mutations, whereas AmoyDx panel can detect 63 types of EGFR mutations at the coding level according to the attached documents15,17. Therefore, EGFR mutations may be detectable in one test but not in the other. However, no reports have investigated the extent to which these two CDx tests cover EGFR mutations in lung cancer specimens.
In this study, we aimed to simulate the differences in EGFR mutation detection at the level of nucleotide changes between the ODxTT and AmoyDx panel and assess the impact on treatment applicability. To this end, we used a dataset obtained from the analysis of an in-house lung cancer panel. These simulation data provide useful insights into whether CDx tests detect actionable EGFR mutations and report therapeutic potential.
We performed targeted sequencing using an in-house lung cancer panel covering the entire exon regions of 53 genes and studied 127 NSCLC patients. In these specimens, we identified 216 EGFR mutations: 167 missense mutations, 45 in-frame insertion/deletion mutations and 4 truncating mutations (Fig. 1A, Supplementary Table 1). The identified mutations were distributed as follows: 4.2% (9/216) in exon 18, 22% (47/216) in exon 19, 13% (28/216) in exon 20, 25% (54/216) in exon 21, and 36% (78/216) in other exons (Supplementary Table 2). The most frequently detected mutations were p.L858R at 23% (49/216) and p.E746_A750del at 14% (31/216) (Fig. 1A).
EGFR mutations targeted by CDx tests. (A) Lollipop plot showing the location of amino acid changes in EGFR mutations identified in the in-house panel. Green circles represent missense mutations, black circles represent truncation mutations, and brown circles represent in-frame mutations. The height corresponds to the number of samples in which EGFR mutations were detected. (B) The heatmap shows a list of EGFR mutations in each sample. The vertical axis represents the samples, and the horizontal axis represents the amino acid changes. The top annotations indicate the exon, mutation type, oncogenicity, targeted mutations for ODxTT and AmoyDx panel, mutations registered as actionable in CIViC, and mutations registered as actionable in OncoKB. (C) The variable tree diagram categorizes the EGFR mutations (total of 216 mutations) into targeted mutations for testing (green layer) and oncogenicity (orange layer).
Using the dataset of EGFR mutations, we performed a simulation to assess the detection rate of the ODxTT and AmoyDx panel. Among the 216 EGFR mutations, 110 (51%) were targeted in both CDx tests, 6 (3%) were specific to ODxTT, and 100 (46%) were not included in the target regions of either CDx test (Fig. 1B and Table 1). There were no mutations that were only detected by the AmoyDx panel. All the mutations detectable by the ODxTT and/or AmoyDx panel were located in exons 18–21 (Fig. 1B). However, the mutations not targeted by either CDx test were predominantly found in exons other than exons 18–21 (78%, 78/100) (Fig. 1B, Supplementary Table 3).
To examine the oncogenicity of EGFR mutations, we referred to a precision oncology knowledge base (OncoKB)18. Among the 110 mutations detectable by both CDx tests, 104 (95%) were classified as oncogenic, 5 (5%) as likely oncogenic, and 1 (1%) as a resistant mutation (Fig. 1C and Table 1). Among the six mutations detectable only by ODxTT, three (50%) were oncogenic, two (33%) were likely oncogenic, and one (17%) was a resistant mutation (Fig. 1C). The six mutations covered by ODxTT but not by the AmoyDx panel were p.E709A (c.2126A > C), p.E709G (c.2126A > G), p.L718Q (c.2153 T > A), p.E746_T751delinsVA (c.2237_2253delAATTAAGAGAAGCAACAinsTTGCT), p.G796S (c.2386G > A), and p.L861R (c.2582 T > G) (Table 2).
The majority of the 100 mutations not included in the target regions of both CDx tests (92/100, 92%) had unknown functional significance (Fig. 1C). However, a small number of mutations were classified as oncogenic (3/100, 3%) or likely oncogenic (3/100, 3%) (Fig. 1C and Table 1). These mutations were p.A750P (c.2248G > C), p.E709_T710delinsD (c.2127_2129delAAC), p.P772_H773insQA (c.2318_2319insCAGGCG), p.R776C (c.2326C > T), p.L858R (c.2573_2574delTGinsGT), and p.A871G (c.2612C > G) (Table 2).
To determine whether the EGFR mutations included in the target regions of the ODxTT and AmoyDx panel corresponded to therapeutic mutations, we referred to the Clinical Interpretation of Variants in Cancer (CIViC) and OncoKB databases18,19. Among a total of 127 cases, 96 (76%) had actionable EGFR mutations, while 31 (24%) had non-actionable mutations (Fig. 2).
Relationship between actionable mutations covered by CDx tests and clinical cases. Variable tree diagram classifying lung cancer patients (total of 127 cases) with EGFR mutations into cases with actionable mutations according to CIViC and OncoKB (purple layer) and cases with targeted mutations for testing (green layer).
Among the 96 cases with actionable mutations, 93 (97%) had mutations detectable by both the ODxTT and AmoyDx panel, 1 (1%) had a mutation detectable only by ODxTT (p.E746_T751delinsVA [c.2237_2253delAATTAAGAGAAGCAACAinsTTGCT] in case ID: P040), and 2 (2%) had mutations not included in the target regions of either test (p.E709_T710delinsD [c.2127_2129delAAC] in case ID: P117 and p.P772_H773insQA [c.2318_2319insCAGGCG] in case ID: P015) (Fig. 2 and Table 2). EGFR p.E709_T710delinsD (c.2127_2129delAAC) in exon 18 is registered in the Catalogue of Somatic Mutations In Cancer (COSMIC; ID: COSV51779132) and the corresponding drug is afatinib with level 3A bases on OncoKB. EGFR p.E709_T710delinsD is classified into P-loop and αC-helix compressing (PACC) subgroups and is sensitive to second-generation TKIs20. The exon 20 insertion mutation p.P772_H773insQA (c.2318_2319insCAGGCG) is considered a rare mutation with no COSMIC registration21, but the corresponding drugs are amivantamab and mobocertinib with level 1 bases on OncoKB. These results suggested that both CDx tests can lead to EGFR TKI treatment for most patients. However, simulation results showed that actionable mutations could not targeted by the two CDx tests at a frequency of 2% (Fig. 2).
In two patients, we observed the presence of EGFR co-mutations, where one mutation was not detected by either CDx test, and the other mutation was detected by both tests and was classified as an actionable mutation (Supplementary Table 4). In case P065, p.L858R (c.2573 T > G)/p.R776C (c.2326C > T) double mutation was identified, while in case P077, p.L858R (c.2573_2574delTGinsGT)/p.T790M (c.2369C > T) double mutation was observed. In these cases, the impact on treatment applicability was not affected because neither one of the detectable mutations represented an actionable alteration. Notably, however, the multi-nucleotide variant (MNV) of p.L858R (c.2573_2574delTGinsGT) that was observed in case P077 was not included in the target regions of both CDx tests. As a result, the therapeutic applicability of these diagnostic tests may be limited when rare EGFR mutations are present in samples.
This study showed whether the ODxTT and AmoyDx panel could detect EGFR mutations from a real-world specimen from patients with lung cancer. Our analysis simulated that among the 216 EGFR mutations, 51% were targeted by both CDx tests, 3% were specific to ODxTT, and 46% were not included in the target regions of either test. All EGFR mutations detected by either of the two tests or by ODxTT were located in exons 18–21 and were functionally relevant mutations. In contrast, the majority of mutations not covered by both CDx tests were located outside exons 18–21 and were functionally unknown mutations. There were actionable EGFR mutations in 96 patients, of which 93 patients (97%) were targeted by both CDx tests. Therefore, the treatment option of EGFR TKIs can be selected for most NSCLC patients by performing ODxTT and AmoyDx panel. In contrast, addressing the forthcoming challenge involves ensuring the identification of the remaining three cases without overlooking them in CDx tests.
There are rare EGFR actionable mutations in NSCLC patients. Notably, this study included the rare EGFR p.L858R mutation caused by an MNV (c.2573_2574delTGinsGT) and this MNV is not targeted by either CDx test. Regarding the MNV, we have previously reported that the Kirsten rat sarcoma viral oncogene homolog (KRAS) p.Q61K (c.180_181delTCinsAA) was detected in colorectal cancer through next-generation sequencing analysis, but not by a real-time PCR based assay22. The AmoyDx panel is based on the amplification refractory mutation system23, which uses 3'-prime mismatches of the nucleotide changes. Therefore, the AmoyDx panel possibly can detect both the single nucleotide variant (c.2573 T > G) and the MNV (c.2573_2574delTGinsGT); however, validation is necessary using samples with the MNV because detailed primer information is not disclosed. Therefore, covering rare EGFR actionable mutations by CDx tests remains a future challenge.
EGFR TKIs are drugs that can be highly effective for patients with EGFR-positive NSCLC. Thus, it is crucial to minimize any potentially missed mutations by the CDx test. In Japan, the ODxTT and AmoyDx panel are the main CDx tests that can analyze multiple genes simultaneously. Either one of these tests is supported by the national health insurance system, but undergoing both tests is not supported. Furthermore, the targeted mutations are different between the two CDx tests, causing concern and frustration when selecting a CDx test. One contributing factor is that the targeted EGFR mutations are different, making it unclear how many EGFR mutations can be reported to patients. In this study, actionable EGFR mutations that could be detected by both CDx tests covered 97% of cases, allowing treatment options to be determined for the majority of patients. However, in 2% of cases, actionable mutations were not detected by either test. The challenge is how to identify these missed cases in first-line treatment. Such discrepancies have also been reported in previous studies24,25. To date, ODxTT has expanded the number of detectable variants included in the report through updates to the analysis program. To reduce the number of missed cases, there is a need to expand the scope of reported rare EGFR mutations in current next generation sequencing-based analyses or to approve newly developed CDx tests26,27.
This study had several limitations. First, this study simulated detectable mutations by the ODxTT and AmoyDx panel using accumulated datasets from our in-house lung cancer panel. Therefore, whether EGFR mutations can actually be detected by these two platforms remains uncertain. Second, an increase in EGFR mutation datasets is needed to obtain more robust results. In particular, rare mutations in EGFR have been reported28; therefore, it is necessary to pay attention to whether they are detected by these CDx platforms. Finally, this cohort includes patients who were treated with EGFR-TKIs and have acquired EGFR resistance mutations. In fact, the ODxTT and AmoyDx panel are used before first-line treatment for NSCLC. These differences may have influenced the EGFR detection rate in this study.
Overall, our findings provide useful information about EGFR detection by the ODxTT and AmoyDx panel. Both CDx tests showed efficacy in detecting clinically relevant mutations and there were small differences in their performance in identifying rare EGFR mutations. This information is valuable for clinicians in selecting the most appropriate diagnostic test based on the specific needs of each patient with NSCLC.
In this study, we selected patients with EGFR-mutated NSCLC who had undergone targeted sequencing with an in-house lung cancer panel (see below). We used datasets from 282 samples (103 tumor tissues, 34 cytological specimens, 18 plasma, and 127 buffy coats) from 127 patients with NSCLC. This included 63 men (50%) and 64 women (50%) with a median age of 69 years old (interquartile range, 63–75). Written informed consent was obtained from all patients. This study was approved by the Institutional Review Board of the Clinical Research and Genome Research Committee at Yamanashi Central Hospital (G-2018-1) and complied with Declaration of Helsinki principles.
Peripheral blood samples were collected and centrifuged to separate buffy coats and plasma. Buffy coats were stored at − 80 °C until DNA extraction. Buffy coat DNA was extracted with the QIAamp DNA Blood Mini QIAcube Kit (Qiagen, Hilden, Germany). The DNA concentration of buffy coats was determined using a NanoDrop 2000 (Thermo Fisher Scientific, Waltham, MA, USA). Plasma DNA was extracted with the MagMax Cell-Free DNA extraction kit on the KingFisher Duo Prime (Thermo Fisher Scientific). Concentration of plasma DNA was determined using the Qubit dsDNA HS Assay Kit and Qubit 3.0 fluorometer (Thermo Fisher Scientific) according to the manufacturer’s instructions.
Tumor tissues were fixed using 10% buffered formalin29. Serial 10 μm sections were prepared from formalin-fixed paraffin-embedded (FFPE) tissues, and sections were stained with hematoxylin–eosin and reviewed by a pathologist to check the tumor area. Laser capture microdissection was performed using an Arcturus XT laser microdissection system (Thermo Fisher Scientific). FFPE DNA was extracted using the QIAamp DNA FFPE Tissue Kit (Qiagen), the GeneRead DNA FFPE Kit (Qiagen), and the MagMAX™ FFPE DNA/RNA Ultra Kit (Thermo Fisher Scientific) according to the manufacturer’s instructions. FFPE DNA concentrations were determined using the Qubit® dsDNA HS Assay Kit on a Qubit Fluorometer 3.0 (Thermo Fisher Scientific).
We used an in-house lung cancer panel targeting 53 genes using IonAmpliseq Designer, as described previously30. Briefly, multiplex PCR was performed using the Ion AmpliSeq Library Kit v2.0 or Ion AmpliSeq Library Kit Plus (Thermo Fisher Scientific). Primers were digested with FuPa reagent and then barcoded using Ion Xpress Barcode Adapters. Purification was performed by Agencourt AMPure XP reagents (Beckman Coulter, Brea, CA, USA) using the KingFisher Duo Prime System (Thermo Fisher Scientific). The library concentration was determined using an Ion Library Quantitation Kit. Emulsion PCR and chip loading were performed on the Ion Chef with the Ion PI Hi-Q Chef Kit. Sequencing was performed using the Ion PI Hi-Q Sequencing Kit on the Ion Proton Sequencer (Thermo Fisher Scientific).
Targeted sequencing was also conducted on the Ion Torrent Genexus System in accordance with the manufacturer’s instructions (Thermo Fisher Scientific). DNA concentrations were diluted to 1.1 ng/µL in nuclease-free water. Amplification of DNA was performed using the aforementioned in-house lung cancer panel. The Ion Torrent Genexus Library Strips and Templating Strips were incubated at room temperature for 30 min before being loaded into the sequencer.
Raw signal data from the sequencing analysis were processed using the standard pipeline in the Torrent Suite Software running on the Torrent Server or in the Genexus Software in the Ion Torrent Genexus System. The data processing pipeline involved signaling processing, base calling, quality score assignment, read alignment, quality control of mapping, and coverage analysis. Following data analysis, the annotation of somatic variants was performed by the Ion Reporter Server System (Thermo Fisher Scientific). To perform tumor–normal pair analysis, we used buffy coat DNA as a normal control for subtraction of germline mutations and to detect somatic variants in tumors. We used the following filtering parameters for variant calling: (i) minimum number of variant allele reads ≥ 10; (ii) coverage depth ≥ 50; (iii) variant allele fraction (VAF) ≥ 0.05; (iv) UCSC Common SNPs = Not In; and (v) Confident Somatic Variants = In.
If the same EGFR mutation was identified in multiple samples from a single patient, duplicate mutations were excluded and not used in the subsequent analysis. The evaluation of EGFR mutations targeted by the ODxTT (Thermo Fisher Scientific) and AmoyDx Pan Lung Cancer PCR Panel (Amoy Diagnostics Co., Ltd., Xiamen, China) was performed according to the attached documents, which were from Thermo Fisher Scientific and Riken Genesis (Tokyo, Japan) as of June 2023 (Supplementary Table 5). Comparisons with corresponding mutations were conducted based on coding information. Mutations that could be detected by both CDx tests were classified as “Both.” Mutations that could only be detected by ODxTT were categorized as “ODxTT only.” Mutations that were not covered by either test were classified as “not targeted.” We also searched the registration of EGFR mutations in COSMIC, version 98 released 23 May 2331.
To evaluate the oncogenicity of mutations, we referred to OncoKB as of June 20, 202318. We searched for the gene name "EGFR" and extracted the mutations under the “Annotated Alterations” tab, specifically focusing on the “Oncogenic” category. If the identified mutation corresponded to EGFR exon 19 in-frame insertions/deletions and exon 20 in-frame insertions, these mutations were classified as “Likely oncogenic”.
To assess the actionable mutations, we searched both the CIViC and OncoKB databases as of June 20, 2023. We downloaded the tab-separated values (.tsv) file of Clinical Evidence Summaries from the CIViC Data Releases (date: March 1, 2023) and selected entries that included “molecular_profile” with “EGFR,” “disease” with “Lung,” and “therapies” with mentions of corresponding drugs (Supplementary Table 6). Additionally, we downloaded the ‘Actionable Genes’ dataset from OncoKB, selecting “EGFR” as the gene and “Non-Small Cell Lung Cancer” as the cancer type (Supplementary Table 7).
Mutations were categorized as follows: if a corresponding drug was mentioned in either CIViC or OncoKB, it was classified as “actionable”; if no mention was found in either database, it was classified as “non-actionable”. Furthermore, in cases where multiple EGFR co-mutations were present in a single patient, if at least one mutation was present in the target lists of both CDx tests, it was categorized as “Both”. If at least one mutation was present in the target list of ODxTT only and did not meet the previous condition, it was classified as “ODxTT only”. If neither condition was met, the case was categorized as “Not targeted”.
Data processing and visualization in R (version 4.1.1) (http://www.r-project.org/) were also performed using ggplot2 (v3.3.5), ggpubr (v0.4.0), dplyr (v1.0.7), tidyr (v1.1.3), scales (v1.2.1), patchwork (v1.1.1), gtsummary (v1.5.2), flextable (v.0 0.7.0), vtree (v.5.6.5), and ComplexHeatmap (v.2.14.0) packages.
The source data underlying figures and tables are available upon reasonable request from the corresponding author.
Youlden, D. R., Cramb, S. M. & Baade, P. D. The international epidemiology of lung cancer: Geographical distribution and secular trends. J. Thorac. Oncol. 3, 819–831. https://doi.org/10.1097/JTO.0b013e31818020eb (2008).
Chan, S. K., Gullick, W. J. & Hill, M. E. Mutations of the epidermal growth factor receptor in non-small cell lung cancer—Search and destroy. Eur. J. Cancer 42, 17–23. https://doi.org/10.1016/j.ejca.2005.07.031 (2006).
Article CAS PubMed Google Scholar
Kosaka, T. et al. Mutations of the epidermal growth factor receptor gene in lung cancer: Biological and clinical implications. Cancer Res. 64, 8919–8923. https://doi.org/10.1158/0008-5472.can-04-2818 (2004).
Article CAS PubMed Google Scholar
Collisson, E. A. et al. Comprehensive molecular profiling of lung adenocarcinoma. Nature 511, 543–550. https://doi.org/10.1038/nature13385 (2014).
Article ADS CAS Google Scholar
Shigematsu, H. et al. Clinical and biological features associated with epidermal growth factor receptor gene mutations in lung cancers. JNCI J. Natl. Cancer Inst. 97, 339–346. https://doi.org/10.1093/jnci/dji055 (2005).
Article CAS PubMed Google Scholar
Hsu, W. H., Yang, J. C., Mok, T. S. & Loong, H. H. Overview of current systemic management of EGFR-mutant NSCLC. Ann. Oncol. 29, i3–i9. https://doi.org/10.1093/annonc/mdx702 (2018).
Lynch, T. J. et al. Activating mutations in the epidermal growth factor receptor underlying responsiveness of non-small-cell lung cancer to Gefitinib. N. Engl. J. Med. 350, 2129–2139. https://doi.org/10.1056/NEJMoa040938 (2004).
Article CAS PubMed Google Scholar
Shepherd, F. A. et al. Erlotinib in previously treated non-small-cell lung cancer. N. Engl. J. Med. 353, 123–132. https://doi.org/10.1056/NEJMoa050753 (2005).
Article CAS PubMed Google Scholar
Sequist, L. V. et al. Phase III Study of Afatinib or Cisplatin Plus Pemetrexed in patients with metastatic lung adenocarcinoma with EGFR mutations. J. Clin. Oncol. 31, 3327–3334. https://doi.org/10.1200/jco.2012.44.2806 (2013).
Article CAS PubMed Google Scholar
Soria, J.-C. et al. Osimertinib in untreated EGFR-mutated advanced non-small-cell lung cancer. N Engl J Med 378, 113–125. https://doi.org/10.1056/NEJMoa1713137 (2017).
Mitsudomi, T. & Yatabe, Y. Epidermal growth factor receptor in relation to tumor development: EGFR gene and cancer. FEBS J 277, 301–308. https://doi.org/10.1111/j.1742-4658.2009.07448.x (2010).
Article CAS PubMed Google Scholar
John, A., Shah, R. A., Wong, W. B., Schneider, C. E. & Alexander, M. Value of precision medicine in advanced non-small cell lung cancer: real-world outcomes associated with the use of companion diagnostics. Oncologist 25, e1743–e1752. https://doi.org/10.1634/theoncologist.2019-0864 (2020).
Article CAS PubMed PubMed Central Google Scholar
Takahashi, T. et al. Real-world study of next-generation sequencing diagnostic biomarker testing for patients with lung cancer in Japan. Cancer Sci. 114, 2524–2533. https://doi.org/10.1111/cas.15752 (2023).
Article CAS PubMed PubMed Central Google Scholar
Sakata, S. et al. Real-world data on NGS using the Oncomine DxTT for detecting genetic alterations in non-small-cell lung cancer: WJOG13019L. Cancer Sci. 113, 221–228. https://doi.org/10.1111/cas.15176 (2022).
Article CAS PubMed Google Scholar
Kunimasa, K. et al. Clinical application of the AMOY 9-in-1 panel to lung cancer patients. Lung Cancer https://doi.org/10.1016/j.lungcan.2023.107190 (2023).
Amemiya, M. S. et al. Actionable driver DNA variants and fusion genes can be detected in archived cytological specimens with the Oncomine Dx target test multi-CDx system in lung cancer. Cancer Cytopathol. 129, 729–738. https://doi.org/10.1002/cncy.22434 (2021).
Article CAS PubMed Google Scholar
Nagakubo, Y. et al. Nucleic acid quality assessment is critical to the success of the oncomine Dx target test for lung cancer. Mol. Diagn Therapy https://doi.org/10.1007/s40291-023-00653-2 (2023).
Chakravarty, D. et al.OncoKB: A precision oncology knowledge base.JCO Precis.Oncol.1, 1–16 (2017).
Griffith, M. et al. CIViC is a community knowledgebase for expert crowdsourcing the clinical interpretation of variants in cancer. Nat. Genet. 49, 170–174. https://doi.org/10.1038/ng.3774 (2017).
Article CAS PubMed PubMed Central Google Scholar
Robichaux, J. P. et al. Structure-based classification predicts drug response in EGFR-mutant NSCLC. Nature 597, 732–737. https://doi.org/10.1038/s41586-021-03898-1 (2021).
Article ADS CAS PubMed PubMed Central Google Scholar
Galli, G. et al. Uncommon mutations in epidermal growth factor receptor and response to first and second generation tyrosine kinase inhibitors: A case series and literature review. Lung Cancer 115, 135–142. https://doi.org/10.1016/j.lungcan.2017.12.002 (2018).
Nagakubo, Y. et al. Accurate detection of KRAS, NRAS and BRAF mutations in metastatic colorectal cancers by bridged nucleic acid-clamp real-time PCR. BMC Med. Genom. 12, 162. https://doi.org/10.1186/s12920-019-0610-8 (2019).
Newton, C. R. et al. Analysis of any point mutation in DNA. The amplification refractory mutation system (ARMS). Nucleic Acids Res. 17, 2503–2516. https://doi.org/10.1093/nar/17.7.2503 (1989).
Article MathSciNet CAS PubMed PubMed Central Google Scholar
Kunimasa, K. et al. Late recurrence of lung adenocarcinoma harboring EGFR exon 20 insertion (A763_Y764insFQEA) mutation successfully treated with osimertinib. Cancer Genet. 256, 57–61. https://doi.org/10.1016/j.cancergen.2021.04.001 (2021).
Article CAS PubMed Google Scholar
Tsuda, T. et al. Rare MYH9-ROS1 fusion gene-positive lung adenocarcinoma showing response to entrectinib treatment: A case study. Case Rep. Oncol. 15, 376–381. https://doi.org/10.1159/000524071 (2022).
Article PubMed PubMed Central Google Scholar
Morikawa, K. et al. A prospective validation study of lung cancer gene panel testing using cytological specimens. Cancers 14, 3784 (2022).
Article CAS PubMed PubMed Central Google Scholar
Kage, H. et al. Clinical utility of Todai OncoPanel in the setting of approved comprehensive cancer genomic profiling tests in Japan. Cancer Sci. 114, 1710–1717. https://doi.org/10.1111/cas.15717 (2023).
Article CAS PubMed PubMed Central Google Scholar
Zhao, W. et al. Rare mutation-dominant compound EGFR-positive NSCLC is associated with enriched kinase domain-resided variants of uncertain significance and poor clinical outcomes. BMC Med. 21, 73. https://doi.org/10.1186/s12916-023-02768-z (2023).
Article CAS PubMed PubMed Central Google Scholar
Amemiya , K. , Hirotsu , Y. , Oyama , T. & Omata , M. Relationship between formalin reagent and success rate of targeted sequencing analysis using formalin fixed paraffin embedded tissues .Clin.Chim.Acta 488, 129–134.https://doi.org/10.1016/j.cca.2018.11.002 (2019).
Article CAS PubMed Google Scholar
Goto, T., Hirotsu, Y., Oyama, T., Amemiya, K. & Omata, M. Analysis of tumor-derived DNA in plasma and bone marrow fluid in lung cancer patients. Med. Oncol. 33, 29. https://doi.org/10.1007/s12032-016-0744-x (2016).
Article CAS PubMed Google Scholar
Tate, J. G. et al. COSMIC: The catalogue of somatic mutations in cancer. Nucleic Acids Res. 47, D941–D947. https://doi.org/10.1093/nar/gky1015 (2019).
Article CAS PubMed Google Scholar
This study was supported by a Grant-in-Aid for the Genome Research Project from Yamanashi Prefecture (to M.O. and Y.H.), the Japan Society for the Promotion of Science (JSPS) KAKENHI Early-Career Scientists JP18K16292 (to Y.H.), a Grant-in-Aid for Scientific Research (B) 20H03668 and 23H02955 (to Y.H.), a Research Grant for Young Scholars (to Y.H.), the YASUDA Medical Foundation (to Y.H.), the Uehara Memorial Foundation (to Y.H.), and Medical Research Grants from the Takeda Science Foundation (to Y.H.) and Kato Memorial Bioscience Foundation (to Y.H.). We thank H. Nikki March, PhD, from Edanz (https://jp.edanz.com/ac) for editing a draft of this manuscript. We also thank Dr. Kei Kunimasa for technical comments on this manuscript.
These authors contributed equally: Yosuke Hirotsu and Takahiro Nakagomi.
Genome Analysis Center, Yamanashi Central Hospital, 1-1-1 Fujimi, Kofu, Yamanashi, 400-8506, Japan
Lung Cancer and Respiratory Disease Center, Yamanashi Central Hospital, 1-1-1 Fujimi, Kofu, Yamanashi, Japan
Takahiro Nakagomi & Taichiro Goto
Division of Genetics and Clinical Laboratory, Yamanashi Central Hospital, 1-1-1 Fujimi, Kofu, Yamanashi, Japan
Department of Gastroenterology, Yamanashi Central Hospital, 1-1-1 Fujimi, Kofu, Yamanashi, Japan
The University of Tokyo, 7-3-1 Hongo, Bunkyo-ku, Tokyo, Japan
You can also search for this author in PubMed Google Scholar
You can also search for this author in PubMed Google Scholar
You can also search for this author in PubMed Google Scholar
You can also search for this author in PubMed Google Scholar
You can also search for this author in PubMed Google Scholar
Y.H.: formal analysis, investigation, data curation, writing—original draft, visualization, and funding acquisition. T.N.: validation, investigation, resources, data curation, writing—review & editing, and project administration. Y.N.: validation and investigation. T.G.: resources and supervision. M.O.: conceptualization, supervision, and writing—review & editing. All authors reviewed the manuscript.
The authors declare no competing interests.
Springer Nature remains neutral with regard to jurisdictional claims in published maps and institutional affiliations.
Open Access This article is licensed under a Creative Commons Attribution 4.0 International License, which permits use, sharing, adaptation, distribution and reproduction in any medium or format, as long as you give appropriate credit to the original author(s) and the source, provide a link to the Creative Commons licence, and indicate if changes were made. The images or other third party material in this article are included in the article's Creative Commons licence, unless indicated otherwise in a credit line to the material. If material is not included in the article's Creative Commons licence and your intended use is not permitted by statutory regulation or exceeds the permitted use, you will need to obtain permission directly from the copyright holder. To view a copy of this licence, visit http://creativecommons.org/licenses/by/4.0/.
Hirotsu , Y. , Nakagomi , T. , Nagakubo , Y. et al.Simulation analysis of EGFR mutation detection: Impact of Oncomine Dx target test and AmoyDx panel on lung cancer treatment decisions.Sci Rep 14, 1594 (2024).https://doi.org/10.1038/s41598-024-52006-6
DOI: https://doi.org/10.1038/s41598-024-52006-6
Anyone you share the following link with will be able to read this content:
Sorry, a shareable link is not currently available for this article.
Provided by the Springer Nature SharedIt content-sharing initiative
By submitting a comment you agree to abide by our Terms and Community Guidelines. If you find something abusive or that does not comply with our terms or guidelines please flag it as inappropriate.
Scientific Reports (Sci Rep) ISSN 2045-2322 (online)
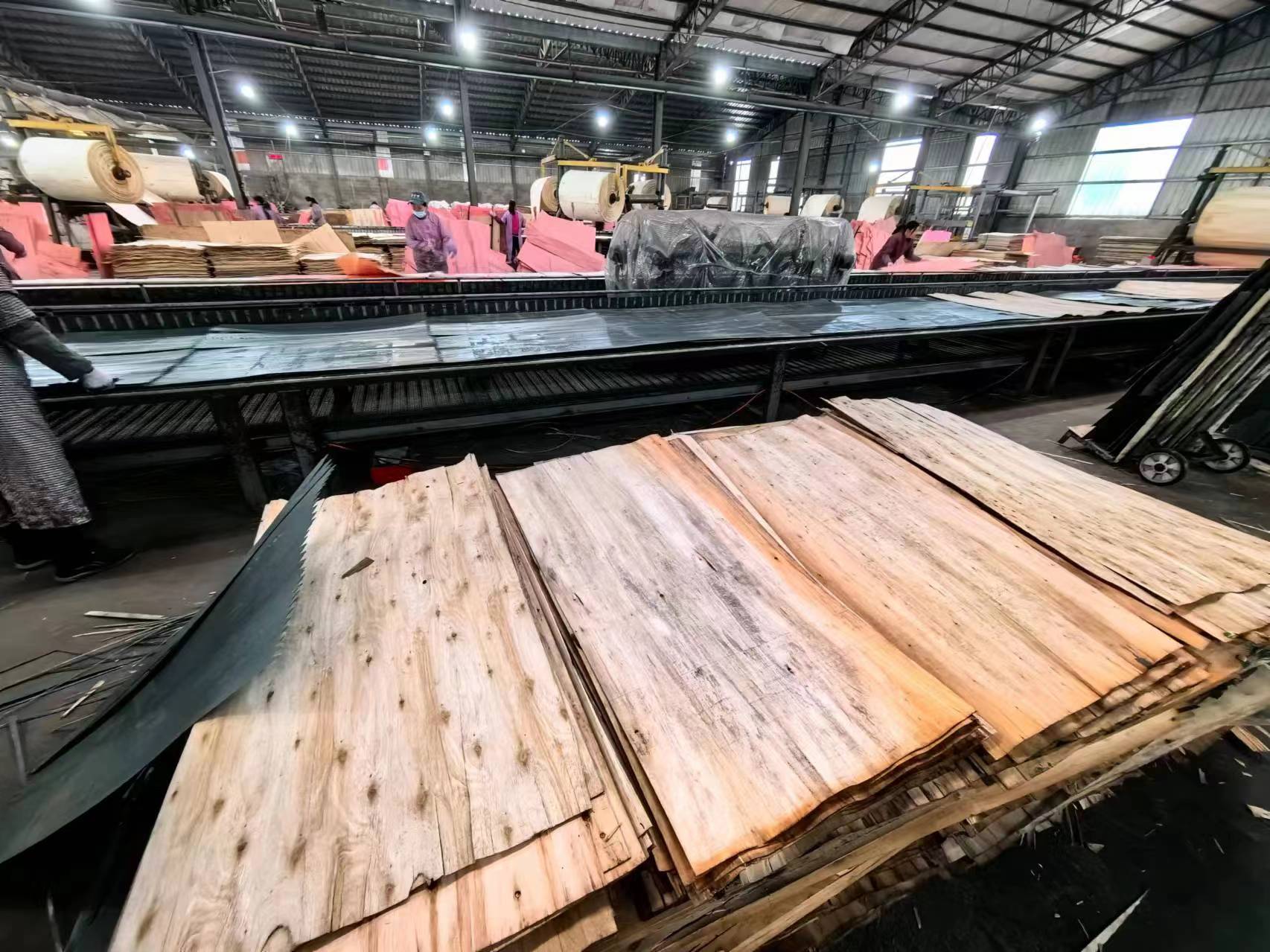
Fire Retardant Plywood Sign up for the Nature Briefing: Cancer newsletter — what matters in cancer research, free to your inbox weekly.