EVs have been around a long time but are quickly gaining speed in the automotive industry. Learn how the long-coming and inevitable shift to electric impacts you.
Whether you want to build your own home theater or just learn more about TVs, displays, projectors, and more, we've got you covered. Dc Mcb Solar
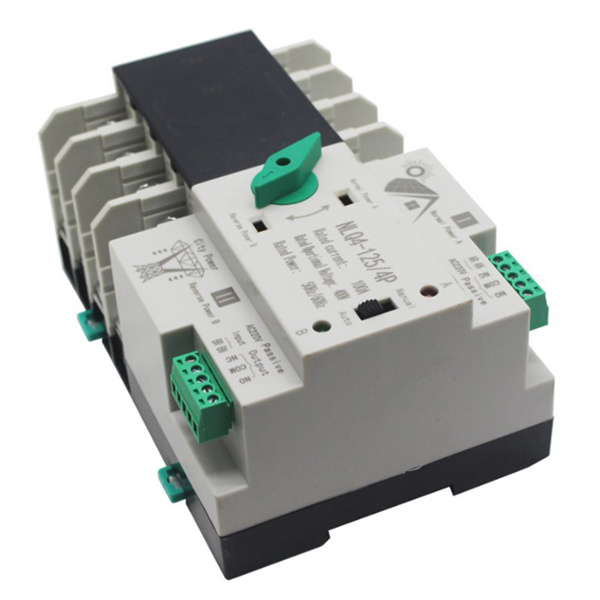
Get tech's top stories in 30 seconds:
Plug in and stay connected while on the road or even a boat
David Beren is a former tech and commerce writer for Lifewire with 10+ years' experience. He has written and managed content for companies like T-Mobile, Sprint, and TracFone Wireless and contributor for sites like WebGeekStuff and MakeTechEasier.
We independently evaluate all recommended products and services. If you click on links we provide, we may receive compensation. Learn more.
The best power inverters should have high wattage, plenty of outlets, resistance to overloads, short circuits, and high temperatures to keep your devices safe. A power inverter can turn DC power into power for AC devices (typical for most consumer electronics).
Our top pick for the category is the KRIEGER 1100-watt inverter. It's one of the world's smallest inverters and powerful enough to handle most small alliances and electronics. The casing is heavy-duty, there's an LCD, and it can manage TVs, gaming consoles, and microwaves with its 2200 watts of peak power.
LCD display is a handy addition
Billed as the world's smallest 1100W power inverter, the Krieger is powerful enough to handle small appliances and electronics. Packed in a heavy-duty aluminum casing durable enough to last for years, the Krieger provides all of its relevant info on an LCD, including output wattage, input voltage, and battery level. It's ideal for keeping power tools charged or powering televisions, gaming consoles, or small appliances such as a microwave (it features 2200 watts of peak power).
Plugging any of those appliances into the KRIEGER is easy, thanks to the two standard AC 12V outlets on dual USB charging ports that push out 2.1A, making it equally suitable for charging smartphones or tablets. KRIEGER's three-year warranty backs it.
Wattage: 1100W | Ports: AC (2), USB (2) | Wave Type: Modified Sine Wave
Produces lower voltage than indicated
BESTEK is one of the most highly regarded and well-known names in the power inverter space, and their 300W product is a standout choice for buyers looking for something small (it's about the length of a smartphone). The BESTEK includes two 110V AC outlets for charging electronics like a laptop and has dual USB charging ports for charging smartphones or tablets.
The included two-foot cigarette adapter plug combines with the BESTEK's small footprint to fit into nearly any vehicle, making it a great choice to pack while camping or on vacation. A built-in 40-amp fuse and the integrated cooling fan help protect both a car and plugged-in devices from overheating, overloading, or overcharging. The robust metal housing ensures the BESTEK can handle a few drops and bumps.
Wattage: 300W | Ports: AC (2), USB (2) | Wave Type: Modified Sine Wave
The AMPEAK 2000-watt power inverter offers a maximum power output of 4000 surge watts. The available three AC outlets and single 2.1A USB outlet will work with everything from cell phones, digital cameras, electrical fans, freezers, floodlights, microwaves, and other electronics you might find in an RV.
To protect against device damage, the AMPEAK adds some welcome protections, including three cooling fans and an audible alarm to alert you to overloads, over-voltage, or overheating. Another significant benefit to the AMPEAK 2000W is the opportunity to connect to a 12V battery system, allowing the AMPEAK to provide additional power for your needs during events such as a hurricane or storm where power outages can be frequent.
Wattage: 2000W | Ports: AC (3), USB (1) | Wave Type: Modified Sine Wave
Smart fan keeps noise to a minimum
Auto-shutdown if battery falls below a certain level
Built-in alarm is incredibly loud
You can never go wrong by having too much power on you while driving across town or the country. The POTEK 500W is an ideal option for buyers looking at power inverters that are appropriately sized for a car and keep the energy flowing to a slew of electronics and hand-held devices. The POTEK 500W and its dual 110V AC outlets and two USB ports will have no problem keeping a laptop, Kindle, iPad, or multiple smartphones going with power to spare.
Keeping all of these charges going simultaneously without causing any damage is the work of the built-in cooling fan that ensures no devices or the POTEK itself will get overheated or overloaded. Measuring 7.1 x 4.1 x 2.2 inches in size, the POTEK fits in most glove compartments or center consoles, which makes it a must-have for anyone who spends a good chunk of time commuting in a car.
Wattage: 500W | Ports: AC (2), USB (2) | Wave Type: Modified Sine Wave
The Renogy 2000W is a jack-of-all-trades pure sine wave power inverter. It's optimized for 12 VDC systems and offers overload protection for DC input and AC output. It protects devices from under-voltage, over-voltage, over-temperature, overload, and short circuits, with LED indicators for each item. High-speed ventilation fans are built into the unit to keep it from overheating, and there's a ground-fault circuit interrupter to prevent short circuits.
The power the Renogy 2000W produces is good enough for tools, fans, lights, and other electronics. As a final selling point, it has a built-in 5V/2.1A USB port and an AC hardwire port to cover all your needs and devices.
Wattage: 2000W | Ports: AC (2), USB (2) | Wave Type: Sine Wave
Variety of safety features to prevent shorts
Can handle computers and other sensitive equipment
Lifespan of fuses is a bit short
The Power TechON 3000 Pure Sine Wave power inverter keeps things powered up while on a boat.
It's equipped with dual AC inputs, one USB port, and a hardware terminal and can reach a maximum of 6000 watts in surge power. The Power TechON 3000 has many protection features to prevent overvoltage or overloaded devices.
It comes with black and red starter cables and a wired remote that can reach up to 15 feet away. Once plugged in, the inverter will have no problem producing power to several devices, including computers, laptops, smartphones, and other handheld electronics, whether you're on the water or docked.
Wattage: 3000W | Ports: AC (2), USB (1) | Wave Type: Sine Wave
The more wattage an inverter has, the more power it can generate. Depending on your use, you may need large loads of power, or your consumption may be minimal. Before you buy a power inverter, consider how you’ll be using the device and try to determine how much power you’ll need. Power inverters vary widely in wattage, from 300W to 3,000W and up. Some can even generate surge power as high as 6,000W.
Most power inverters have two standard AC outlets for various electronic devices. Other models offer additional AC outlets and often USB ports to charge all of your devices—from laptops to fans and flood flights.
If you need a power inverter that can withstand the elements, look for a durable device with features like an aluminum casing. Many inverters also have extra protection features to prevent overvoltage or overloaded devices. Still feeling insecure? Look for an inverter with a warranty for even more protection.
A power inverter transforms direct current (DC) power into alternating current (AC) power that powers many common devices and appliances. Because DC power is derived from batteries, an inverter can provide a great way to run or charge your devices on the go.
Inverters rated for 450 watts and under usually include a cable that allows you to connect them to your vehicle by way of a cigarette lighter outlet. Larger inverters (500W and up) typically need to be wired up directly to the battery.
The best way to utilize multiple batteries with an inverter is to chain together identical 12-volt batteries in parallel. This will dramatically increase the amount of time between recharging the batteries that power your devices.
Get the Latest Tech News Delivered Every Day
Hit Refresh on Your Tech News
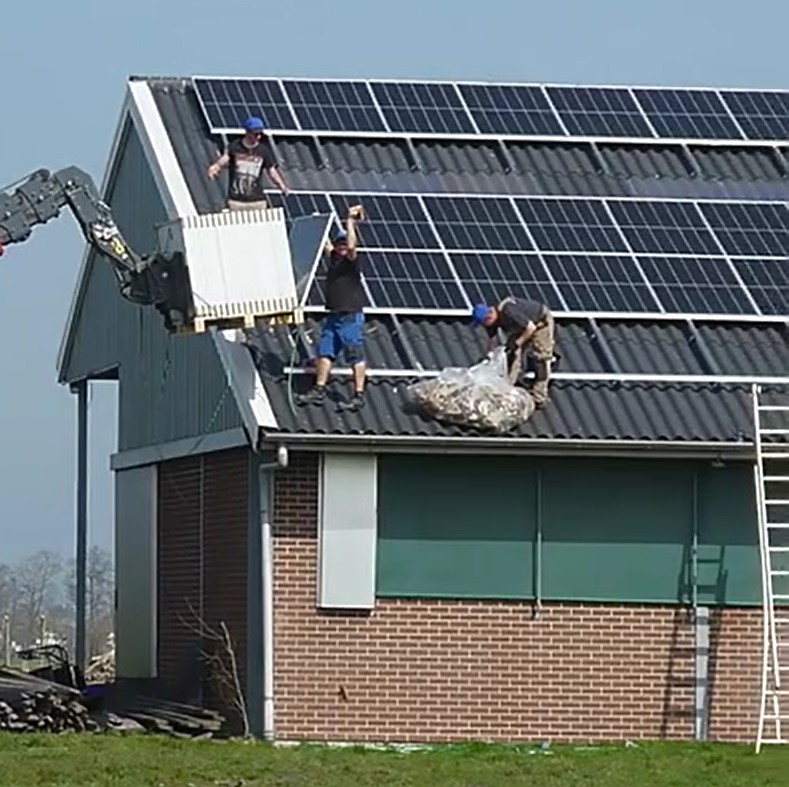
Electric Car Charger For Home By clicking “Accept All Cookies”, you agree to the storing of cookies on your device to enhance site navigation, analyze site usage, and assist in our marketing efforts.