Thank you for visiting nature.com. You are using a browser version with limited support for CSS. To obtain the best experience, we recommend you use a more up to date browser (or turn off compatibility mode in Internet Explorer). In the meantime, to ensure continued support, we are displaying the site without styles and JavaScript.
Scientific Reports volume 14, Article number: 7381 (2024 ) Cite this article Hydrolyzed Fish And Seaweed Fertilizer

The aim of the present work is to biosynthesize Chitosan nanoparticles (CTNp) using tea (Camellia sinensis) extract, with potent antimicrobial properties towards phytopathogens of rice. Preliminary chemical analysis of the extract showed that they contain carbohydrate as major compound and uronic acid indicating the nature of acidic polysaccharide. The structure of the isolated polysaccharide was analyzed through FTIR and 1H NMR. The CTNp was prepared by the addition of isolated tea polysaccharides to chitosan solution. The structure and size of the CTNp was determined through FTIR and DLS analyses. The surface morphology and size of the CTNp was analysed by SEM and HRTEM. The crystalinity nature of the synthesized nanoparticle was identified by XRD analysis. The CTNp exhibited the antimicrobial properties against the most devastating pathogens of rice viz., Pyricularia grisea, Xanthomonas oryzae under in vitro condition. CTNp also suppressed the blast and blight disease of rice under the detached leaf assay. These results suggest that the biosynthesized CTNp can be used to control the most devastating pathogens of rice.
Global demand for food is predicted to increase by 70% in the next few decades which alarms the importance of sustainable agricultural production1. Rice (Oryza sativa L.) is one of the principal cereal crop which represents a staple food worldwide. Rice cultivation is adapted to a wide range of agro-climatic zones, hence FAO (Food and Agricultural Organization) regarded it as a strategic crop for food security2,3. However, rice production is constrained due to various diseases such as blast, leaf blight, sheath blight, sheath rot caused by phytopathogens4. Among them, Pyricularia grisea and Xanthomonas oryzae are the major destructive pathogens of rice, which cause blast, leaf blight (BLB) disease respectively and leads to severe yield losses5,6. They are widely prevalent among various rice varieties worldwide and both the pathogens infect the rice crop at all stages of growth, thus affecting the nutrient quality and quantity in terms of yield5. To control phytopathogens and to boost-up production, farmers use different types of chemical fertilizers and fungicides in paddy field7. The emerging plant pathogen resistance towards commonly used fungicides as well as potential threats of these agrochemicals in the ecosystem warrants effective control of plant pathogens through ecofriendly approach. Therefore, novel approaches that are ecofriendly and sustainable are necessary to control these phytopathogens.
Nanotechnology is one of the key technologies that promise to advance traditional agricultural practices for sustainable plant disease management8,9,10,11. The application of nanotechnology in agriculture for increased crop production was encouraged by FAO12,13,14. Nanomaterials have the potential to minimize the chemical input and protect crops from devastating phytopathogens15,16,17,18. Nanoparticles have been integrated into plant disease management approach as bactericides/fungicides to enhance plant and soil health19,20,21. Nanoparticles prepared from biopolymers have received much attention due to their biodegradability, biocompatibility and non-toxicity22,23,24,25. Chitosan, a cationic biopolymer obtained from crustaceans and its nanoparticles are very well documented for their broad spectrum antimicrobial properties towards phytopathogens26,27,28,29 and they also induce defense in various crops30,31,32. Chitosan nanoparticles are generally prepared chemically (besides physical method) by ionotrophic gelation using sodium tripolyphosphate (TPP). Though TPP is considered as safe it results in nanoparticles with variation in size, stability and biological property which affects its wide application in agriculture27,33. To minimize the size variation and to increase the stability, it is of necessary to identify an alternate green approach to prepare chitosan nanoparticles. Green synthesis offers advantage over traditional chemical and physical methods as it is environmentally acceptable and economically feasible method.
Tea (Camellia sinensis L.) and its extracts have been used as medicinal and dietary supplements to induce immunity, in many countries. Tea extracts are rich in bioactive compounds, such as polyphenols, anionic polysaccharides etc.34,35,36,37. In recent years, tea polysaccharides (TPS) received more attention due to their various biological functions38. This study reports the phytofabrication of chitosan nanoparticles through green approach using tea leaf extract (tea polysaccharides) as an alternative ecofriendly procedure. The green synthesized chitosan nanoparticles were characterized and evaluated for its antimicrobial property against phytopathogens of rice under in vitro condition.
Tea polysaccharides were isolated from tea leaves using standard procedure and purified through DEAE-cellulose chromatography. The carbohydrate and protein content present in TPS isolated from leaves of C. sinensis was 89.4 ± 0.36% and 1.8 ± 0.3% of the total dry weight respectively. The uronic acid content was found to be 9 ± 0.2% indicating the nature of acidic polysaccharide. Preliminary TLC analysis indicates the presence of galactose, mannose, glucose, arabinose, xylose, and rhamnose in the polysaccharide (TPS) which is in accord with previous report39. FTIR spectrum indicates the presence of characteristic absorption peaks of polysaccharides (Fig. 1). A strong and broad absorption peak around 3441 cm−1, 2985 cm−1 due to O–H and C–H stretching vibrations respectively (Fig. 1) were observed. The absorption peak at 1638 cm-1 indicates C=O stretch and the presence of a carbonyl group of acidic polysaccharide40. The peak at 1453 cm−1 and 1405 cm−1 were attributed to C–H stretch of polysaccharides. The absorption peak at 1253 cm−1 due to C–O stretch indicates the existence of uronic acid characteristics of TPS. The glycosidic linkage stretch was observed at 1046 cm−1. The absorption peak at 877 cm−1 indicates α-glycosidic linkage in polysaccharide chains37.
FTIR spectrum of Tea polysaccharide.
To confirm the presence of polysaccharides in the tea extract, iH NMR was done. The 1H NMR analysis showed a set of wide and complex signals present between δH 3.1–5.2 ppm (Fig. 2) was characteristic of the typical polysaccharide signal41. Signals between 3.9–4.6 and 5.0–5.2 ppm confirmed the existence of both α and β configuration of saccharide residues40,42 which supports the result obtained from FTIR. These data suggest that the isolated product from tea leaf extract mainly composed of polysaccharides (TPS).
1H NMR spectrum of isolated TPS.
Of the various concentrations tried, TPS prepared at 2.5 mg/ml displayed the best result in forming the opalescent solution. The opalescent appearance observed after the addition of 27–30 ml of TPS to 100 ml of chitosan solution at the given temperature and pH. The TPS acts as a precursor/reducing agent and aided the formation of nanoparticles. It might be possible that anionic tea polysaccharide attaches to cationic NH2 groups of chitosan and convert them to chitosan nanoparticles. This bioreduction method involves single-step process and is eco-friendly, non-toxic. The formation of nanoparticles was primarily analyzed through UV–visible spectrophotometer which showed a single peak at 373 nm indicating the biofabrication of chitosan tea nanoparticles (CTNp). Green fabrication of chitosan nanoparticles using Lavendula angustifolia with antibiofilm activity has been reported43.
FTIR analysis (Fig. 3) showed the presence of peak at 3436 cm−1 due to overlap of the OH and NH stretching. The typical peak at 2927 cm−1 due to C–H stretch indicates the structure of chitosan. The peak at 1644 cm−1 showed the presence of a carbonyl bond of amide group. The peak at 1319 cm−1 (N=O stretch), 1156 cm−1 indicates the binding of chitosan to TPS (Fig. 3). The presence of uronic acids was observed at 1260 cm−1. The glycosidic linkage was observed at 1029 cm−1 (C–O stretch). FTIR analysis indicates the structural stability of chitosan during the bioconversion of chitosan nanoparticles.
FTIR spectrum of biosynthesized CTNp.
The diameter of the nanoparticle as measured by zetasizer was 118.7 nm with PDI value of 0.36 (Fig. 4a). This indicates the monodisperse nature of CTNp in aqueous solution which is vital for the biological activity44. The addition of acidic polysaccharides to chitosan solution aids the formation of chitosan nanoparticles, which might be due to simple electrostatic interaction. Surface charge identified through zeta potential analysis is an important parameter to evaluate the nanoparticle stability in suspension. For a physically stable nanoparticle suspension, a minimum zeta potential of ± 30 mV is required45,46. A high positive or negative zeta potential resists aggregation of nanoparticles in suspension47 due to the repulsion and confers stability. The zeta potential of the biosynthesized CTNp was observed to be + 33.1 mV (Fig. 4b). Higher positive ZP (+ 33.1 mV) of biosynthesized chitosan nanoparticles could exhibit substantial electrostatic repulsion between nanoparticles and thus prevents aggregation in aqueous phase. These results suggest that phytofabrication using tea extract yields monodispersed highly stable CTNp as compared to other methods27,48.
Dynamic Light Scattersing (a), Zeta Potential (b) of CTNp.
HRTEM analysis showed well dispersed, spherical shaped nanoparticles with a size range between 25 and 40 nm (Fig. 5a). SEM micrograph revealed that the surface of nanoparticles was observed to be smooth and spherical (Fig. 5b). The size difference between TEM and DLS analyses may be due to different measurement conditions viz., dry and hydrodynamic state respectively involved in these techniques. The XRD analysis showed no peaks in the diffractogram (Fig. 6) implying the amorphous nature of nanoparticles, which augment the sorption properties of the materials.
HRTEM image (a) and SEM image (b) of CTNp.
Biosynthesized CTNp and its individual bulk products (chitosan, TPS) used for synthesis of nanoparticles were evaluated for its antimicrobial potential against X. oryzae and P. grisea, the most devastating rice pathogens. Biosynthesized CTNp showed significant difference in growth inhibition of X. oryzae when compared to control, chitosan and TPS (Fig. 7a,c) under in vitro condition. Substantial inhibition (more than 90%) of hyphal growth and spore germination of P. grisea after exposure to biosynthesized CTNp (Fig. 7b,d) was observed. The bulk chitosan and TPS were found to be less effective in inhibiting the hyphal growth and spore germination of P. grisea under the same condition. These results indicated that biosynthesized CTNp was more effective in controlling both P. grisea and X. oryzae under in vitro condition. This may be due to its size/surface charge which makes CTNp as highly permeable towards the biological membrane. It is possible that the small size of CTNp makes easy entry through the fungal cell wall and binds to their DNA, proteins and inhibits the synthesis of mRNA26. The high positive surface charge of CTNp might interact with negatively charged biological membrane/cell wall of phytopathogens tested and alter the membrane permeability their by inhibits/control their growth. This was in accord with previous reports29,49,50,51.
Antimicrobial activity of CTNp against X. oryzae (a, c) and P. grisea (b, d). C-Control, Ch-Chitosan, TPS-Tea polysaccharide, CTNp-Chitosan tea nanoparticle, A-Standard Antibiotic.
The detached leaf method provides the in vivo atmosphere to the interacting phytopathogens and therefore exhibit similar results as of whole plants48. In chitosan, TPS treated leaves, the blast disease appeared after 4 days of infection whereas in control the symptom appeared after 2 days and progress further. Nearly 50% blast disease suppression was observed in chitosan and TPS treated leaves when compared to untreated infected control. In CTNp treated leaves, complete suppression of blast disease was observed in the test period (Figs. 8a, 9a). Likewise, the disease suppression was also observed for BLB disease in CTNp treated leaves when compared to its bulk counterparts (Figs. 8b, 9b). These results substantiate the biocidal property of biosynthesized CTNp. To our knowledge, this is the first report on plant disease suppression activity of TPS and CTNp. Inhibition of phytopathogenic fungus, Botrytis cinerea by green synthesized chitosan nanoparticles were reported recently52. CTNp can enter the plant system easily due to its small size through stomata, wounds, trichomes etc.53,54. Stomata serve as an efficient route for nanoparticle uptake47,49. Rice being a monocot has stomata on both sides55 which might have enhanced the rapid uptake of chitosan nanoparticles. Once it enters inside it might activate the defense response, their by control the growth of invading phytopathogens besides its biocidal property. In addition, the higher number of surface functional groups present in CTNp aids easy interaction with plant cells. The outcome of this work suggests that CTNp can be green synthesized using tea extract and can be used in the agricultural sector for disease management. Further work is necessary to evaluate the biosynthesized CTNp in protecting rice crops under field conditions.
Blast (a) and Leaf Blight (b) disease suppression on detached leaf of O. sativa. C-Control, Ch-Chitosan, TPS-Tea polysaccharide, CTNp-Chitosan tea nanoparticle.
% infection of Blast (a) and Blight (b) disease on detached rice leaf. C-Control, Ch-Chitosan, TPS-Tea polysaccharide, CTNp-Chitosan tea nanoparticle.
This study reports the biosynthesis of chitosan nanoparticles using the extract of C. sinensis. The tea extract was partially purified through DEAE cellulose column chromatography. Preliminary chemical analysis indicates it as acidic polysaccharide. The structure was analyzed by FTIR and 1H NMR. The biosynthesized chitosan nanoparticles were characterized through FTIR, HRTEM, SEM, DLS and XRD analyses. The zeta potential of the biosynthesized CTNp was observed to be + 33.1 mV, indicating its stability in aqueous solution. CTNp showed inhibitory activity against the most devastating rice pathogens viz., P. grisea and X. oryzae under in vitro condition. Detached leaf method showed suppression of the blast, blight diseases of rice and suggests that CTNp can be used to control rice phytopathogens. However, this requires field evaluation to establish.
Tea polysaccharide was extracted following the procedure of34. Tea (Camellia sinensis L.) was purchased (black tea; includes both leaves and buds) from the local market, extracted (1 g/50 ml) with water at 85 °C for 3 h and cooled to room temperature. The extract was concentrated to 5 ml using rotary vapor with continuous rotation in a water bath set at 37 °C and it was further extracted twice with equal volume of chloroform: n-butanol (4:1, v/v) to remove the contaminating proteins. To the aqueous phase, two volumes of ice cold ethanol (95%) was added, kept at 4 °C overnight and centrifuged at 12,000 G for 15 min (Eppendorf 5415R, Germany) to precipitate the polysaccharides. The precipitate was washed twice with ethanol, dissolved in sterile distilled water, dialyzed against distilled water for 24 h at 4 °C and freeze dried. The extract (0.5 g/ml) was then passed through DEAE-cellulose column chromatography (30 × 2.5 cm diameter; at room temperature) pre-equilibrated with sterile distilled water. The sample was sequentially (3 ml/fraction) eluted using distilled water with 0.1–0.5 M NaCl gradient solutions. Fractions were tested for the presence of carbohydrate by the Dubios method. Fractions showing peaks were pooled together dialyzed against distilled water (to remove the salt) at 4 °C for 24 h, freeze dried and used for characterization.
Total polysaccharide content was quantified by the phenol–sulphuric acid method following Dubios et al.56 using galactose as a standard. Protein content was analyzed by Bradford57 method using BSA fraction V as a standard. Uronic acid was determined based on Blumenkrantz and Asboe-Hansen58 method. The crude polysaccharide (1 mg) was hydrolyzed with 200 µl of 3 M trifluroacetic acid at 120 °C for 4 h. The hydrolyzates were centrifuged and separated (10 µl) on silica gel coated TLC (0.2 mm thickness) plates along with standards (Sigma Chem. Co., USA) using n-butanol: pyridine: water (50:28:32) as solvent system. The plates were air dried, sprayed with aniline phthalate reagent and Rf values were calculated. FTIR analysis was performed in Nicolet 560 FTIR spectrophotometer in a frequency range between 400 and 4000 cm−1 using the KBR pellet method. The 1H NMR spectra were recorded on a Bruker DRX-500 MHZ spectrophotometer using D2O as solvent system.
Chitosan (85% acetylation; molecular weight 27 kDa; 250 mg/100 ml) dissolved in 0.1% acetic acid and pH was adjusted to 4.2. The tea extracts (TPS) were prepared separately (0.5, 1.0. 1.5, 2.0, 2.5, 3.0 mg/ml) in sterile distilled water and added to chitosan solution drop by drop under stirring at room temperature till the appearance of opalescent solution. Further, it was allowed under magnetic stirring for 2–3 h and the colloidal solution was centrifuged at 15,000 G for 30 min. The precipitate was washed with 70% (v/v) ethanol and freeze dried.
UV–visible spectra were recorded (200–400 nm) using Shimadzu UV–visible 1800 Spectrophotometer for preliminary confirmation of nanoparticle formation. FTIR was performed by KBr pellet method in the region of 400–4000 cm−1, to identify the functional groups in the nanoparticles using Nicolet 560 FTIR spectrophotometer. Particle size, surface charge and poly dispersity index (PDI) were analyzed by DLS using Zetasizer (Malvern, UK) at 25 °C in triplicate. The nanoparticle size and surface morphology were examined by HRTEM (JOEL model, 1200 Ex) and SEM (Zeiss, Evo 18, Germany) respectively. X-ray diffraction studies were performed in X-ray diffractometer (Rigaku Ultima III XRD) with a CuKα1 radiation to determine the structure of the sample. The X-ray source was operated at 40 kV and 40 mA. Diffraction intensity was measured in the reflection mode at a scanning rate of 2/min for 2θ = 10°–70°.
The biosynthesized chitosan nanoparticles were evaluated for its antimicrobial property against the most devastating rice pathogens viz., Pyricularia grisea and Xanthomonas oryzae under in vitro condition.
The mycelial disc (7 mm diameter) was removed from the actively growing end of seven day old culture of P. grisea, placed upside down position in a petri plate containing potato dextrose agar (PDA) medium. To its opposite, a sterile filter disc (6 mm diameter) impregnated with (100 µg/100 µl) chitosan, TPS, CTNp was placed separately and incubated at 25 ± 2 °C for 10 days. For control, filter discs were added with sterile distilled water. Experiments were done in triplicate and repeated thrice. % of inhibition was calculated as follows:
For spore assay, spore suspension (1 × 105 spores/ml) of P. grisea was prepared in sterile distilled water from 10 days old cultures grown in potato dextrose agar medium. Czepak’s Dox broth (half strength) medium amended with (0.1%, w/v) chitosan, TPS, CTNp were prepared separately. To this, the spore suspension (5 ml/50 ml medium) was added and incubated in an orbital shaker at 27 °C with 120 rpm for 7 days. Optical readings were taken at 600 nm at every 12 h interval up to 7 days. The experiments were repeated thrice with triplicates.
Antibacterial activity (X. oryzae) of synthesized chitosan nanoparticle (CTNp) was done by disc diffusion method using Muller-Hinton agar (MHA) medium. Actively growing (over night grown) culture of X. oryzae (100 µl) was added in the centre of the petri plate and uniformly spread over the medium. Sterile filter disc (6 mm diameter) impregnated with (100 µg/100 µl) chitosan, TPS, CTNp was placed in the medium along with standard antibiotics. For control, sterile distilled water was added to the disc. The petri plates were incubated at 37 °C for 24 h. For each sample, five plates were used and repeated thrice. % of inhibition was calculated using the formula:
For spectrophotometrical assay, nutrient broth (half strength) medium amended with (0.1%, w/v) chitosan, TPS, CTNp were prepared separately. To this, active X. oryzae culture (1 ml/10 ml medium) was added and incubated in an orbital shaker at 37 °C with 120 rpm for 48 h. Optical readings were taken at 600 nm at every 8 h interval up to 48 h. The experiments were repeated thrice with triplicates.
To evaluate the effect of CTNp on blast and leaf blight disease suppression, detached leaf bioassay was done. Collection of plant material, complied with relevant institutional, national, and international guidelines and legislation. Rice plants were grown in pots under in vitro condition. Leaves from 30 d old rice plants were washed thoroughly in running tap water followed by sterile distilled water. The leaves were treated (both abaxial and adaxial surfaces) with 0.1% (w/v) chitosan, TPS, CTNp separately (1 ml/leaf) using a paint brush, placed in sterile petri plate and incubated at 25 ± 2 °C for 24 h. For control, water was used. After 24 h, the control and treated leaves were inoculated with (1 ml/leaf) P. grisea spore suspensions (1 × 105 spores/ml) using a paint brush. The plates were maintained at 25 ± 2 °C with 90% humidity for 10–14 days to monitor the blast disease development.
Another set of experiments was performed using X. oryzae. X. oryzae was grown in nutrient broth over night and the culture was harvested (1 O.D at 600 nm). Treated and control leaves (as above) were infected with X. oryzae (1 ml/leaf) and the plates were incubated at 25 ± 2 °C. The leaf blight disease development was monitored for the next 14 days.
All the data were subjected to one-way analysis of variance (ANOVA) to determine the significance of individual differences in p < 0.01 and 0.05 levels. All statistical analysis was conducted using SPSS 21 software support version 5.0.
All data generated during this study are included in this article.
Pawlak, K. & Kolodziejczak, M. The role of agriculture in ensuring food security in developing countries: Considerations in the context of the problem of Sustainable food production. Sustainability 12, 5488 (2020).
Montano, P. F. et al. Plant growth promotion in cereal and leguminous agricultural important plants: From microorganism capacity to crop production. Microbiol. Res. 169, 325–336 (2014).
Senguttuvel, P. et al. Evaluation of genotype by environment interaction and adaptability in lowland irrigated rice hybrids for grain yield under high temperature. Sci. Rep. 11, 15825 (2021).
Article ADS CAS PubMed PubMed Central Google Scholar
Rangarajan, S., Sleena, L. M., Vasudevan, P. & Nair, S. Biological suppression of rice disease by Pseudomonas spp. under saline soil conditions. Plant Soil 251, 73–82 (2003).
Yasmin, A. et al. Biocontrol of bacterial leaf blight of rice profiling of secondary metabolites produced by rhizospheric Pseudomonas aeruginosa BRp3. Front. Microbiol. 8, 1895 (2017).
Article PubMed PubMed Central Google Scholar
Abi, A. E., Chai, Q. & Coulter, J. A. Rice blast: A disease with implications for global food security. Agronomy 9, 451 (2019).
Dineshkumar, R., Kumaravel, R., Gopalsamy, J., Sikder, M. N. A. & Sampathkumar, P. Microalgae as bio-fertilizers for rice growth and seed yield productivity. Waste Biomass Valoriz. 9, 793–800 (2018).
Parisi, C., Vigani, M. & Rodriguez-Cerezo, E. Agricultural nanotechnologies: What are the current possibilities?. Nano Today 10, 124–127 (2015).
Elmer, W. H. & White, J. C. Role of nanotechnology in plant pathology. Ann. Rev. Phytopathool. 56, 111 (2018).
Hofmann, T. G. V. et al. Technology readiness and overcoming barriers to sustainably implement nanotechnology enabled plant agriculture. Nat. Food 1, 416–425 (2020).
Mali, S. C., Raj, S. & Trivedi, R. Nanotechnology a novel approach to enhance crop productivity. Biochem. Biophys. Rep. 24, 100821 (2020).
Maulin, F. N. et al. Chitosan based agronanofungicides as a sustainable alternative in the basel stem rot disease management. J Agric. Food Chem. 68, 4305–4314 (2020).
Gogos, A. M., Knauer, K. & Bucheli, T. D. Nanomaterials in plant protection and fertilization: Current state, foreseen applications and research priorities. J Agric. Food Chem. 60, 9781–9792 (2012).
Article CAS PubMed Google Scholar
Asadishad, B. et al. Application of agricultural soils with metal nanoparticles: Effects on soil enzyme activity and microbial community composition. Environ. Sci. Technol. 52, 1908–1918 (2018).
Article ADS CAS PubMed Google Scholar
Prasad, R., Bhattacharya, A. & Nguyen, Q. D. Nanotechnology in sustainable agriculture: Recent developments. Challenges Persp. Front. Microbiol. 8, 1014 (2017).
Rodregues, S. M. et al. Nanotechnology for sustainable food production: Promising opportunities and scientific challenges. Environ. Sci. Nano 4, 767–781 (2017).
Lowry, G. V., Avellan, A. & Gilbertson, L. M. Opportunities and challenges for nanotechnology in the agri-tech revolution. Nat. Nanotechnol. 14, 517–522 (2019).
Article ADS CAS PubMed Google Scholar
Divya, K., Thambi, M., Vijayan, S., Varghese, S. & Jisha, M. S. Induction of defense response in Oryza sativa against Rhizoctonia solani by chitosan nanoparticles. Microb. Pathogen. 149, 104525 (2020).
Siddaiah, C. N. et al. Chitosan nanoparticles having high degree of acetylation induce resistance against pearl millet downy mildew through nitric oxide generation. Sci. Rep. 8, 2485 (2018).
Article ADS PubMed PubMed Central Google Scholar
Chen, J. et al. Comparative study on the fungicidal activity of metallic MgO nanoparticles and macroscale MgO against soilborne fungal phytopathogens. Front. Microbiol. 11, 365 (2020).
Article PubMed PubMed Central Google Scholar
Swain, H. et al. Seed biopriming with Trichoderma strains isolated from tree bark improves plant growth, antioxidative defense system in rice and enhance straw degradation capacity. Front. Microbiol. 12, 633881 (2021).
Article PubMed PubMed Central Google Scholar
Kashyap, P. L., Xiang, X. & Heiden, P. Chitosan nanoparticle based delivery systems for sustainable agriculture. Int. J. Biol. Macromol. 77, 36–51 (2015).
Article CAS PubMed Google Scholar
Azmana, M. et al. A review on chitosan and chitosan based bionanocomposites promising matierla for combating global issues and its application. Int. J. Biol. Macromol. 185, 832–848 (2021).
Article CAS PubMed Google Scholar
El Naggar, N. E., Saber, I. A. W., Zweil, A. M. & Bashir, S. I. An innovative green synthesis approach of chitosan nanoparticles and their inhibitory activity against phytopathogenic Botrytis cinerea on strawberry leaves. Sci. Rep. 12, 3515 (2022).
Article ADS PubMed PubMed Central Google Scholar
Prajapati, D. et al. Chitosan nanomaterials: A prelim of next-generation fertilizers; exisiting and future prospects. Carbohyd. Polym. 288, 119356 (2022).
Hadwiger, L. A. Multiple effects of chitosan on plant systems: Solid science or hype. Plant Sci. 208, 42–49 (2013).
Article CAS PubMed Google Scholar
Saharan, V. et al. Synthesis and in vitro antifungal efficacy of Cu-chitosn nanoparticles against pathogenic fungi of tomato. Int. J Biol. Macromol. 75, 346–353 (2015).
Article CAS PubMed Google Scholar
Liu, Y. et al. Chitosan and oligochitosan enhance ginger (Zingiber officinale Roscoe) resistance to rhizome rot caused by Fusarium oxysporum in storage. Carbohydr. Polym. 151, 474–479 (2016).
Article CAS PubMed Google Scholar
Kheiri, A., Jorf, S. M., Malihipour, A., Saremi, H. & Nikkhah, M. Application of chitosan and chitosan nanoparticles for the control of Fusarium head blight of wheat (Fusarium graminearum) in vitro and greenhouse. Int. J. Biol. Macromol. 93, 1261–1272 (2016).
Article CAS PubMed Google Scholar
Saharan, V. et al. Cu-chitosan nanoparticle mediated sustainable approach to enhance seedling growth in maize by mobilizing reserved food. J. Agric. Food Chem. 64, 6148–6155 (2016).
Article CAS PubMed Google Scholar
Divya, K., Smitha, V. & Jisha, M. S. Antifungal, antioxidant and cytotoxic activities of chitosan nanoparticles and its use as an edible coating on vegetables. Int. J. Biol. Macromol. 114, 572–577 (2018).
Article CAS PubMed Google Scholar
Jogaiah, S., Satapute, P., Britto, S. D., Konappa, N. & Udayashankar, A. C. Exogeneous priming of chitosan induces upregulation of phytohormones and resistance against cucumber powdery mildew disease is correlated with localized biosynthesis of defense enzymes. Int. J. Biol. Macromol. 162, 1825–1838 (2020).
Article CAS PubMed Google Scholar
Yu, J. et al. Current trends and challenges in the synthesis and applications of chitosan based nanocomposites for plants: A review. Carbohyd. Polym. 261, 117904 (2021).
Scoparo, C. T. et al. Polysaccharides from green and black teas and their protective effect against murine sepsis. Food Res. Int. 53, 780–785 (2013).
Li, X., Sun, C., Luo, L. & He, Y. Determination of tea polyphenols content by infrared spectroscopy coupled with iPLS and random frog techniques. Comput. Electron. Agric. 112, 28–35 (2015).
Shi, L. Bioactivities, isolation and purification methods of polysaccharides from natural products: A review. Int. J. Biol. Macromol. 92, 37–48 (2016).
Article CAS PubMed PubMed Central Google Scholar
Du, L. L. et al. Tea polysaccharides and their bioactivities. Molecules 21, 1449 (2016).
Article PubMed PubMed Central Google Scholar
Chen, G., Yuan, Q., Saeeduddin, M., Ou, S. & Zeng, X. Recent advances in tea polysaccharides: Extraction, purification, physiochemical characterization and bioactivities. Carbohydr. Polym. 153, 663–678 (2016).
Article CAS PubMed Google Scholar
Chen, C. et al. Effects of tea polysaccharides in combination with polyphenols on dextran sodium sulphate induced colitis in mice. Food Chem. 13, 100190 (2022).
Chen, G. et al. Structural characterization and immunostimulatory activity of heteropolysaccharides from fuzhuan brick tea. J Agric. Food Chem. 69, 1368–1378 (2021).
Article CAS PubMed Google Scholar
Wang, I., Liu, H. & Kim, C. Structure, characterization and antioxidant activity of polysaccharides from Chinese quince seed meal. Food Chem. 234, 314–322 (2017).
Article CAS PubMed Google Scholar
Peng, Z., Mingyong, X., Shaoping, N. & Xiaoru, W. Primary structure and configuration of tea polysaccharide. Sci. China Ser. C Life Sci. 47, 416–424 (2004).
El-Naggar, N. E. A., Eltarahony, M., Hafez, E. E. & Bashir, S. I. Green fabrication of chitosan nanoparticles using Lavendula angustifolia, optimization, characterization and in-vitro antibiofilm activity. Sci. Rep. 13, 11127 (2023).
Article ADS CAS PubMed PubMed Central Google Scholar
Van, S. N., Minh, H. D. & Ahn, D. N. Study on chitosan nanoparticles on biophysical characteristics and growth of Robusta coffee in green house. Biocatal. Agric. Biotechnol. 2, 289–294 (2013).
Du, W. L., Niu, S. S., Xu, Y., Xu, Z. R. & Fan, C. L. Antibacterial activity of chitosan nanoparticles loaded with various metal ions. Carbohydr. Polym. 25, 385–389 (2009).
Zain, M. N., Stapley, A. G. F. & Sharma, G. Green synthesis of silver and copper nanoparticles using ascorbic acid and chitosan for antibacterial applications. Carbohydr. Polym. 112, 195–202 (2014).
Article CAS PubMed Google Scholar
Ali, S. W., Rajendran, S. & Joshi, M. Synthesis and characterization of chitosan and silver loaded chitosan nanoparticles. Carbohydr. Polym. 83, 436–446 (2011).
Chandra, S. et al. Chitosan nanoparticles: A positive modulator of innate immune responses in plants. Sci. Rep. 5, 15195 (2015).
Article ADS CAS PubMed PubMed Central Google Scholar
Ashok Kumar, D., Palanichamy, V. & Selvaraj, M. P. Green aynthesis of silver nanoparticles using Alternanthera dentate leaf extract at room temperature and their antimicrobial activity. Spectrochem. Acta Part A Mol. Biomol. Spectrosc. 127, 168–171 (2014).
Madhumitha, G., Elango, G. & Selvaraj, M. P. Bio-functionalized doped silver nanoparticles and its antimicrobial studies. J. Sol-Gel Sci. Technol. 73, 476–483 (2015).
Rajeshkumar, S., Yadav, K., Sridharan, M. & Selvaraj, M. P. Nano-silver: An overview of shape, size-controlled synthesis and their antibacterial property. High Energy Chem. 57, 205–216 (2023).
El-Naggar, N. E. A., Saber, W. I., Zweil, A. M. & Bashir, S. I. An innovative green synthesis approach of chitosan nanoparticles and their inhibitory activity against phytopathogenic Botrytis cinerea on strawberry leaves. Sci. Rep. 12, 1–20 (2022).
Fernandez, V. & Eichert, T. Uptake of hydrophilic solutes through plant leaves: Current state of knowledge and perspectives of foliar fertilization. Crit. Rev. Plant Sci. 28, 36–68 (2009).
Su, Y. et al. Delivery, Uptake, fate and transport of engineered nanoparticles in plants: A critical review and data analysis. Environ. Sci. Nano 6, 2311–2331 (2019).
Willmer, C., Fricker, M. (1996).Stomata.
Dubois, M., Gilles, K. A., Hamilton, J. K., Rebers, P. A. & Smith, F. Colorimetric method for determination of sugars and related substances. Anal. Chem. 28, 350–356 (1956).
Bradford, M. M. A rapid and sensitive method for the quantitation of microgram quantities of protein utilizing the principle of protein-dye binding. Anal. Biochem. 72, 248–254 (1976).
Article CAS PubMed Google Scholar
Blumenkrantz, N. & Asboe-Hansen, A. New method for quantitative determination of uronic acids. Anal. Biochem. 54, 484–489 (1973).
Article CAS PubMed Google Scholar
The Authors thank IITM, Chennai for NMR analysis.
This work was supported by RUSA-2.0, MoE, Government of India. RVB thanks DST-INSPIRE and MG thanks RUSA 2.0 for Fellowship. SM thanks Bharathidasan University for Fellowship.
Department of Botany, School of Life Sciences, Bharathidasan University, Tiruchirappalli, Tamil Nadu, 620024, India
M. Sathiyabama, RV Boomija, S. Muthukumar, M. Gandhi, S. Salma, T. Kokila Prinsha & B. Rengasamy
You can also search for this author in PubMed Google Scholar
You can also search for this author in PubMed Google Scholar
You can also search for this author in PubMed Google Scholar
You can also search for this author in PubMed Google Scholar
You can also search for this author in PubMed Google Scholar
You can also search for this author in PubMed Google Scholar
You can also search for this author in PubMed Google Scholar
M. Sathiyabama Conceived, designed the study and wrote the original draft.. R. V. Boomija performed formal analysis and revised the manuscript. S. Muthukumar perfomed Investigation M. Gandhi and B. Rengasamy performed formal analysis. and S. Salma and T. Kokila Prinsha carried statistical analysis. All authors read and approved the final manuscript. All authors consent to submit the manuscript for publication.
The authors declare no competing interests.
Springer Nature remains neutral with regard to jurisdictional claims in published maps and institutional affiliations.
Open Access This article is licensed under a Creative Commons Attribution 4.0 International License, which permits use, sharing, adaptation, distribution and reproduction in any medium or format, as long as you give appropriate credit to the original author(s) and the source, provide a link to the Creative Commons licence, and indicate if changes were made. The images or other third party material in this article are included in the article's Creative Commons licence, unless indicated otherwise in a credit line to the material. If material is not included in the article's Creative Commons licence and your intended use is not permitted by statutory regulation or exceeds the permitted use, you will need to obtain permission directly from the copyright holder. To view a copy of this licence, visit http://creativecommons.org/licenses/by/4.0/.
Sathiyabama, M., Boomija, R.V., Muthukumar, S. et al. Green synthesis of chitosan nanoparticles using tea extract and its antimicrobial activity against economically important phytopathogens of rice. Sci Rep 14, 7381 (2024). https://doi.org/10.1038/s41598-024-58066-y
DOI: https://doi.org/10.1038/s41598-024-58066-y
Anyone you share the following link with will be able to read this content:
Sorry, a shareable link is not currently available for this article.
Provided by the Springer Nature SharedIt content-sharing initiative
By submitting a comment you agree to abide by our Terms and Community Guidelines. If you find something abusive or that does not comply with our terms or guidelines please flag it as inappropriate.
Scientific Reports (Sci Rep) ISSN 2045-2322 (online)
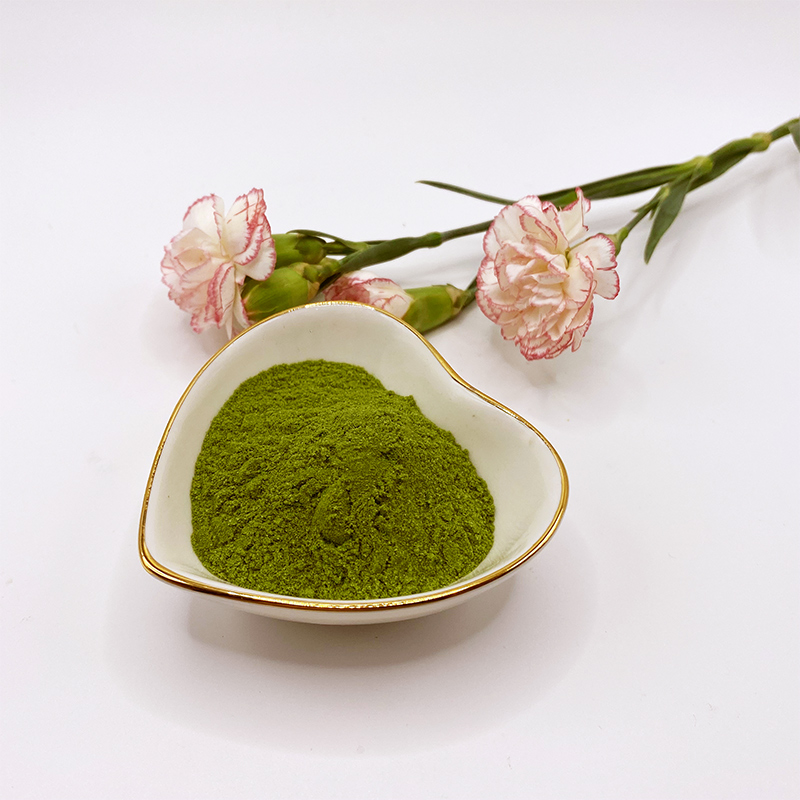
Protein Performance Fertilizer Sign up for the Nature Briefing: Microbiology newsletter — what matters in microbiology research, free to your inbox weekly.