Thank you for visiting nature.com. You are using a browser version with limited support for CSS. To obtain the best experience, we recommend you use a more up to date browser (or turn off compatibility mode in Internet Explorer). In the meantime, to ensure continued support, we are displaying the site without styles and JavaScript.
Scientific Reports volume 13, Article number: 22866 (2023 ) Cite this article 2-Fluorophenylacetone

An Author Correction to this article was published on 30 January 2024
This article has been updated
Chlorine dioxide is a powerful disinfectant with strong antibacterial properties. We conducted a study at different sites of the Lebanese American University Medical Center-Rizk Hospital to determine the efficacy of the ECOM air mask in decreasing the particle load. Air cultures were obtained from three different locations, namely the patients’ elevator, visitors’ elevator and mobile clinic and the number of colonies grown on each type of agar was determined. We also measured particle counts at the three sites both at baseline and after placement of the ECOM air mask. After 7 days of ECOM air mask use, the numbers of colonies grown on all types of media was decreased by 20–100% versus the baseline values. The counts of particles of different diameters (0.3, 0.5 and 5 µm) were decreased at all three sampled sites. This study highlighted the efficacy of the ECOM air mask. The utility of the gaseous form of ClO2 as an antiseptic in the hospital setting appears promising.
Chlorine dioxide (ClO2) is a powerful disinfectant that disrupts protein synthesis and cell membranes and a strong antioxidant that inhibits microbial growth. ClO2 has demonstrated antiviral, antibacterial and antifungal properties in vitro. It is produced by an acid–base reaction or through the use of an electrolytic method1. ClO2 can be used in an aqueous or gaseous state. As a gas, it has a yellow to reddish-yellow colour at room temperature and it has proven sporicidal effects at concentrations of 10–40 mg/L and contact times of at least 30 min on metal, plastic and glass surfaces2. In one study, ClO2 exhibited strong antibacterial properties attributable to its protein-denaturing ability, which is important for disturbing spore viability and/or integrity3. In another study, gaseous ClO2 at an extremely low concentration prevented influenza a virus infection in mice caused by aerosolised particles4.
The maximum residual disinfectant level of ClO2 is 0.8 mg/L according to the disinfectants and disinfection by-products rule. The ClO2 permissible exposure limits defined by the Occupational Safety and Health Administration are 0.1 ppm and 0.3 mg/m3 for general industry1. Even with a low concentration of chlorine dioxide, it is proven effective against micro-organisms5.
The COVID19 pandemic is considered one of the most challenging healthcare crises of the twenty-first century. The rapid and global spread of the disease both jeopardised global health and imposed socioeconomic challenges6. In Lebanon, our ‘Emergency Risk Management Programme’ plan proved inadequate to tackle infectious disease pandemics and the healthcare system was already overloaded prior to the onset of the pandemic, as the country featured the highest ratio of refugees per capita globally. Therefore, the COVID19 pandemic had serious consequences on the healthcare system and the economy7. Chlorine dioxide was proven to be an effective disinfectant during the COVID-19 pandemic5.
Infection control practices should ensure high standard of hygiene at the hospital level for patients and staff safety. In addition, antimicrobial resistance (AMR) is a worldwide rising concern requiring mitigation measures at the level of infection control. According to the Infectious Diseases Society of America, AMR represents one of the biggest threats to human health, as patients infected with multidrug-resistant (MDR) organisms have worse prognoses. Typically, MDR bacteria are more common in the healthcare setting and all efforts should be exerted to prevent their spread to the community8. A study conducted at Wolaita Sodo University Teaching and Referral Hospital revealed that aerosol transmission can contribute to the persistence of bacterial pathogens in the hospital environment and the rise of various healthcare-associated infections. The study additionally identified a high prevalence of airborne bacteria of clinical concern, such as Staphylococcus aureus, Enterococcus, Acinetobacter, Pseudomonas aeruginosa and Escherichia coli, which contributed to a high prevalence of post-surgical site and respiratory tract infections in the study area9. MDR bacteria have been a major concern among patients with COVID-19.
Our study is novel. No previous studies were conducted about the gaseous ClO2 use in a hospital setting. The ES-010 ECOM air mask is a device that emits 0.017–0.031 ppm of ClO2.
Therefore, we examined the efficacy of the gaseous form of ClO2 in killing bacteria and fungi. The aim of this study was to determine the efficacy of the ECOM air mask in decreasing the load of particles in air and the number of bacterial and fungal colonies grown on different types of agar from air samples at the Lebanese American University Medical Center-Rizk Hospital.
To perform this experiment, MacConkey agar (MAC) was used to isolate gram-negative bacteria, trypticase soy agar (TSA) and Luria–Bertani agar (LB) were used to culture different types of microorganisms and Sabouraud agar (Sab) was used to grow pathogenic and non-pathogenic fungi. Samples were collected from the patients’ elevator, visitors’ elevator and mobile clinic, one sample from each location. Prior to placement of the ECOM air mask, the baseline level of microbes was recorded by taking air cultures using SPIN AIR V2 (absorbs 100 L of air in 1 min) on all four types of agar. SPIN AIR V2 is a portable device, that can retrieve accurate volumes of air by forcing them, and after incubation, colony enumeration is done. The ECOM air mask was placed in the middle of each sampling area and samples were taken again after 1 week. The agar plates were incubated at 37 °C for 18 h and the number of colonies that grew on each medium was counted.
To detect the antibacterial and antifungal activity of 0.017–0.031 ppm ClO2 emitted from the ES-010 ECOM air mask, we measured the particle count at the three aforementioned locations (patient elevator, visitor elevator, mobile clinic). First, baseline samples (T0) were obtained, followed by placement of the ECOM air mask. In both elevators, samples were taken 2, 4, 5, 6, 8, 20 and 25 days after placing the ECOM air mask. For the mobile clinic, samples were taken 10, 20 and 24 h and 4 and 15 days after placing the ECOM air mask. The particles were counted using the TSI AeroTrak® 9303 Handheld Particle Counter, which measures all particles based on a set diameter. The TSI AeroTrak® 9303 Handheld Particle Counter is a lightweight plastic device used for particle measurements. The instrument can report up to three particle sizes simultaneously.
As shown in Table 1, After 7 days of ECOM air mask use, the numbers of colonies grown on all types of agar decreased by 20–100% versus the initial values.
The counts of particles of three different diameters (0.3, 0.5 and 5 µm) were recorded at baseline and at several subsequent time points, as in Tables 2, 3, 4.
In the mobile clinic, a major decrease in the particle count was detected within a few hours after mask placement and this decrease was maintained until day 4. However, this decrease was not sustained on day 15, excluding the large particle count, which was decreased by 49%, as in Table 2 and Fig. 1.
Particle count measurements after the placement of the ECOM air mask at the mobile clinic. (A) 0.3 µm. (B) 0.5 µm. (C) 5 µm.
In the visitors’ elevator, seen in Table 3 and Fig. 2 there were sustained decreases in the small (48%) and medium particle counts (43%), whereas the large particle count remained unchanged.
Particle count measurements after the placement of the ECOM air mask at the visitors elevator. (A) 0.3 µm. (B) 0.5 µm. (C) 5 µm.
Similarly, in the patients’ elevator, there were sustained decreases in the small (50%) and medium particle counts (45%), whereas the large particle count remained unchanged as shown in Table 4 and Fig. 3.
Particle count measurements after the placement of the ECOM air mask at the patients elevator. (A) 0.3 µm. (B) 0.5 µm. (C) 5 µm.
Our experiment demonstrated the efficacy of the ECOM air mask in killing microbial pathogens at three locations and decreasing the particle load in air.
The antimicrobial properties of ClO2 were confirmed in this study. According to one study, these effects are attributable to its ability to denature proteins3. In fact, another study demonstrated that ClO2 is a size-selective antimicrobial agent. It can kill micro-sized organisms quickly, but it is incapable of causing measurable damage to much larger organisms because of its inability to penetrate deeply into living tissue. This might render ClO2 relatively safe for human use as an antiseptic10. ClO2 shares an antiseptic mechanism with natural antiseptics such as hypochlorous and hypoiodous acids. Neutrophil granulocytes use these hypohalous acids to kill bacteria after phagocytosis. ClO2 and hypohalous acids target and attack sulfhydryl groups, which are important for the life processes of all living systems. This explains the inability of bacteria to develop resistance against ClO211. In addition, one study demonstrated the antibacterial effect of ClO2 on MDR bacteria12.
The antiviral effect of the gaseous form of ClO2 against RNA and DNA viruses has been reported13. Some studies suggested that this virucidal effect is attributable to the denaturing effect of ClO2 on amino acids such as cysteine, tyrosine and tryptophan in viral protein capsids14. However, because ClO2 exerted a virucidal effect on non-enveloped viruses, it could have other mechanisms of action that require further investigation13. The gaseous form of ClO2 also has antifungal properties and it inactivates mould and bacterial colonies15. Its sporicidal effect is attributable to its ability to affect cell membrane integrity and inhibit germination16.
Seven days after starting the experiment in this study, decreased colony counts were detected on all agar plates for samples obtained from the patients’ elevator and visitors’ elevator. Regarding mobile clinic samples, a decreased colony count was detected on LB and TSA agar plates, whereas small counts were sustained on Sab and MAC agar plates, which might be attributable to contamination.
Regarding the particle count measurement, a decrease of up to 50% was documented for most of the air samples collected. The increased particle count in the mobile clinic can be explained by the fact that the exposure of this area to outdoor settings is significant, whereas in indoor areas such as elevators, the particle count was considerably lower.
The utility of the gaseous form of ClO2 as an antiseptic in the hospital setting appears promising. The presence of ClO2 in the air reduces the number of pathogens present. The use of the ECOM air mask in the hospital setting can disinfect the air, and consequently ensure better hygiene and infection control. Our study demonstrated its efficacy as disinfectant in real life setting. Further studies are required to investigate the antiviral, antifungal and sporicidal effects of ClO2 in the hospital setting.
In the era of artificial intelligence, technology can complement the use of chlorine dioxide in hospital setting by developing predictive models, ensuring real time monitoring and control, and performing automated data analysis.
The first limitation in that our study was conducted only in one center and in specific limited sites. Multicenter studies should be performed in the future in different sites to elaborate and establish guideline for the use of such modalities.
Another limitation is that all the study sites were open areas which might have affected the particle count and culture. However, this reflects the real life setting.
This study highlighted the efficacy of the ECOM air mask in decreasing particle and microbial counts at different hospital sites. The novelty of this study brings an innovative way of use of the gaseous form of ClO2, shown to be an effective antiseptic in hospital setting. However, further studies are needed with more data to support these results and optimize its use.
All data generated or analyzed during this study are included in this published article.
A Correction to this paper has been published: https://doi.org/10.1038/s41598-024-53034-y
Ma, J. W. et al. Efficacy and safety evaluation of a chlorine dioxide solution. Int. J. Environ. Res. Public Health 14(3), 329. https://doi.org/10.3390/ijerph14030329 (2017).
Article CAS PubMed PubMed Central Google Scholar
Morino, H., Fukuda, T., Miura, T. & Shibata, T. Effect of low-concentration chlorine dioxide gas against bacteria and viruses on a glass surface in wet environments. Lett. Appl. Microbiol. 53(6), 628–634. https://doi.org/10.1111/j.1472-765X.2011.03156.x (2011).
Article CAS PubMed PubMed Central Google Scholar
Rastogi, V. K. et al. Systematic evaluation of the efficacy of chlorine dioxide in decontamination of building interior surfaces contaminated with anthrax spores. Appl. Environ. Microbiol. 76(10), 3343–3351. https://doi.org/10.1128/AEM.02668-09 (2010).
Article ADS CAS PubMed PubMed Central Google Scholar
Ogata, N. & Shibata, T. Protective effect of low-concentration chlorine dioxide gas against influenza A virus infection. J. Gen. Virol. 89(1), 60–67. https://doi.org/10.1099/vir.0.83393-0 (2008).
Article CAS PubMed Google Scholar
Jefri, U. H. N. M. et al. A systematic review on chlorine dioxide as a disinfectant. J. Med. Life. 15(3), 313–318. https://doi.org/10.25122/jml-2021-0180 (2022).
Article PubMed PubMed Central Google Scholar
Chakraborty, I. & Maity, P. COVID-19 outbreak: Migration, effects on society, global environment and prevention. Sci. Total Env. 728, 138882. https://doi.org/10.1016/j.scitotenv.2020.138882 (2020).
Bizri, A. R., Khachfe, H. H., Fares, M. Y. & Musharrafieh, U. COVID-19 pandemic: An insult over injury for Lebanon. J. Commun. Health 46(3), 487–493. https://doi.org/10.1007/s10900-020-00884-y (2021).
van Duin, D. & Paterson, D. L. Multidrug-resistant bacteria in the community: Trends and lessons learned. Infect. Dis. Clin. North Am. 30(2), 377–390. https://doi.org/10.1016/j.idc.2016.02.004 (2016).
Article PubMed PubMed Central Google Scholar
Solomon, F. B., Wadilo, F. W., Arota, A. A. & Abraham, Y. L. Antibiotic resistant airborne bacteria and their multidrug resistance pattern at university teaching referral hospital in South Ethiopia. Ann. Clin. Microbiol. Antimicrob. 16(1), 29. https://doi.org/10.1186/s12941-017-0204-2 (2017).
Article CAS PubMed PubMed Central Google Scholar
Noszticzius, Z. et al. Chlorine dioxide is a size-selective antimicrobial agent. PLoS ONE 8(11), e79157. https://doi.org/10.1371/journal.pone.0079157 (2013).
Article ADS CAS PubMed PubMed Central Google Scholar
Smit, M. J. & Anderson, R. Biochemical mechanisms of hydrogen peroxide- and hypochlorous acid-mediated inhibition of human mononuclear leukocyte functions in vitro: Protection and reversal by anti-oxidants. Agents Actions 36(1–2), 58–65. https://doi.org/10.1007/BF01991229 (1992).
Article CAS PubMed Google Scholar
Hinenoya, A. et al. Chlorine dioxide is a better disinfectant than sodium hypochlorite against multi-drug resistant Staphylococcus aureus, Pseudomonas aeruginosa, and Acinetobacter baumannii. Jpn. J. Infect. Dis. 68(4), 276–279. https://doi.org/10.7883/yoken.JJID.2014.294 (2015).
Article CAS PubMed Google Scholar
Sanekata, T. et al. Evaluation of the antiviral activity of chlorine dioxide and sodium hypochlorite against feline calicivirus, human influenza virus, measles virus, canine distemper virus, human herpesvirus, human adenovirus, canine adenovirus and canine parvovirus. Biocontrol Sci. 15(2), 45–49. https://doi.org/10.4265/bio.15.45 (2010).
Article CAS PubMed Google Scholar
Ogata, N. Inactivation of influenza virus haemagglutinin by chlorine dioxide: Oxidation of the conserved tryptophan 153 residue in the receptor-binding site. J. Gener. Virol. 93(12), 2558–2563. https://doi.org/10.1099/vir.0.044263-0 (2012).
Wilson, S. C. et al. Effect of chlorine dioxide gas on fungi and mycotoxins associated with sick building syndrome. Appl. Env. Microbiol. 71(9), 5399–5403. https://doi.org/10.1128/AEM.71.9.5399-5403.2005 (2005).
Article ADS CAS Google Scholar
Young, S. B. & Setlow, P. Mechanisms of killing of Bacillus subtilis spores by hypochlorite and chlorine dioxide. J. Appl. Microbiol. 95(1), 54–67. https://doi.org/10.1046/j.1365-2672.2003.01960.x (2003).
Article CAS PubMed Google Scholar
This work received no specific grant from any funding agency in the public, commercial or not-for-profit sectors.
Division of Emergency, Department of Internal Medicine, School of Medicine, Lebanese American University, Beirut, Lebanon
Division of Infectious Diseases, Department of Internal Medicine, School of Medicine, Lebanese American University, Beirut, Lebanon
Ahmad Mahdi & Rola Husni
Center for Infectious Diseases Research, American University of Beirut, Beirut, Lebanon
Antoine Abou Fayad, Ahmad Sleiman & Ghassan M. Matar
Infection Control Program, Lebanese American University Medical Center, Beirut, Lebanon
Sanaa Zoghbi & Tarek Madani
Lebanese American University-Rizk Hospital, Beirut, Lebanon
You can also search for this author in PubMed Google Scholar
You can also search for this author in PubMed Google Scholar
You can also search for this author in PubMed Google Scholar
You can also search for this author in PubMed Google Scholar
You can also search for this author in PubMed Google Scholar
You can also search for this author in PubMed Google Scholar
You can also search for this author in PubMed Google Scholar
You can also search for this author in PubMed Google Scholar
Conceptualization M.H., A.M., A..F, S.A., G.M., S.Z., T.M., R.H.; methodology, M.H., A.M., R.H.; formal analysis, M.H., A.M., R.H.; investigation, M.H., AM, AF, SA, GM, SZ, TM, RH.; resources, MH, AM, AF, SA, GM, SZ, TM, RH; data curation, MH, AM, AF, SA, GM, SZ, TM, RH; writing—original draft preparation, M.H., A.M., A.F., S.A., G.M., S.Z., T.M., R.H.; writing—review and editing, M.H., A.M., R.H.; supervision, R.H.; project administration, R.H. All authors have read and agreed to the published version of the manuscript.
The authors declare no competing interests.
Springer Nature remains neutral with regard to jurisdictional claims in published maps and institutional affiliations.
The original online version of this Article was revised: The original version of this Article contained an error in the name of author Ahmad Sleiman, which was incorrectly given as Sleiman Ahmad.
Open Access This article is licensed under a Creative Commons Attribution 4.0 International License, which permits use, sharing, adaptation, distribution and reproduction in any medium or format, as long as you give appropriate credit to the original author(s) and the source, provide a link to the Creative Commons licence, and indicate if changes were made. The images or other third party material in this article are included in the article's Creative Commons licence, unless indicated otherwise in a credit line to the material. If material is not included in the article's Creative Commons licence and your intended use is not permitted by statutory regulation or exceeds the permitted use, you will need to obtain permission directly from the copyright holder. To view a copy of this licence, visit http://creativecommons.org/licenses/by/4.0/.
Helou, M., Mahdi, A., Abou Fayad, A. et al. Antimicrobial effects of chlorine dioxide in a hospital setting. Sci Rep 13, 22866 (2023). https://doi.org/10.1038/s41598-023-49997-z
DOI: https://doi.org/10.1038/s41598-023-49997-z
Anyone you share the following link with will be able to read this content:
Sorry, a shareable link is not currently available for this article.
Provided by the Springer Nature SharedIt content-sharing initiative
By submitting a comment you agree to abide by our Terms and Community Guidelines. If you find something abusive or that does not comply with our terms or guidelines please flag it as inappropriate.
Scientific Reports (Sci Rep) ISSN 2045-2322 (online)
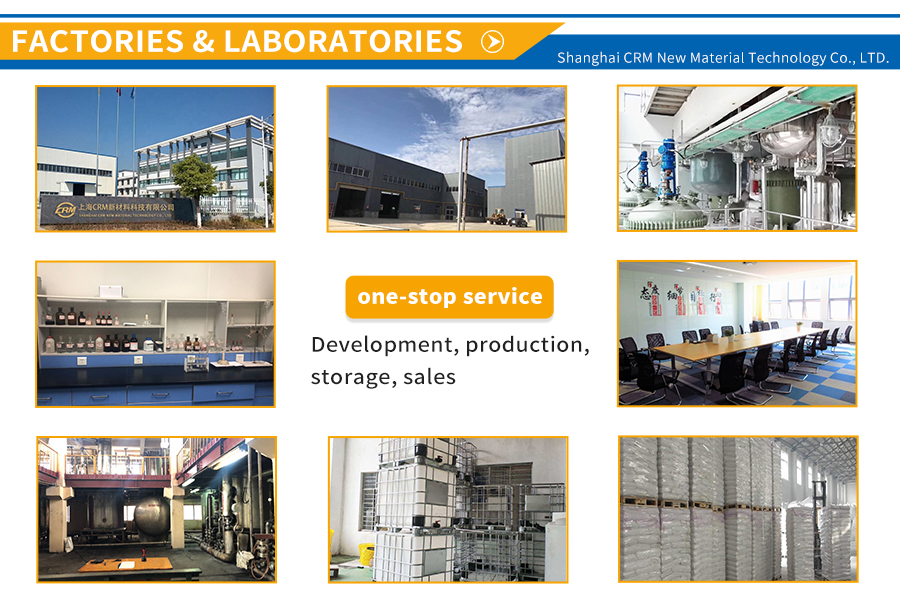
4'-Chloropropiophenone Sign up for the Nature Briefing newsletter — what matters in science, free to your inbox daily.