Thank you for visiting nature.com. You are using a browser version with limited support for CSS. To obtain the best experience, we recommend you use a more up to date browser (or turn off compatibility mode in Internet Explorer). In the meantime, to ensure continued support, we are displaying the site without styles and JavaScript.
Scientific Reports volume 14, Article number: 12117 (2024 ) Cite this article Pcr Tubes With Cap
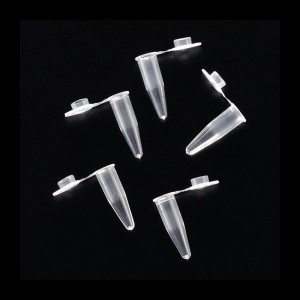
The implementation of the sterile insect technique against Aedes albopictus relies on many parameters, in particular on the success of the sterilization of males to be released into the target area in overflooding numbers to mate with wild females. Achieving consistent sterility levels requires efficient and standardized irradiation protocols. Here, we assessed the effects of exposure environment, density of pupae, irradiation dose, quantity of water and location in the canister on the induced sterility of male pupae. We found that the irradiation of 2000 pupae in 130 ml of water and with a dose of 40 Gy was the best combination of factors to reliably sterilize male pupae with the specific irradiator used in our control program, allowing the sterilization of 14000 pupae per exposure cycle. The location in the canister had no effect on induced sterility. The results reported here allowed the standardization and optimization of irradiation protocols for a Sterile Insect Technique program to control Ae. albopictus on Reunion Island, which required the production of more than 300,000 sterile males per week.
The Sterile Insect Technique (SIT) is a biological and species-specific method of insect pest control. It relies on releasing continuous and overflooding numbers of sexually competitive sterile insects, usually males, into a wild population of the same species. When wild females mate with sterile males, their reproduction is blocked, thus suppressing or even eradicating the target population over successive generations1. Over the last decades, successful SIT programs have suppressed populations of various pests of veterinary and agricultural importance, such as fruit flies, tsetse flies, screw worms and moths2. However, the success of the SIT against mosquitoes, a medically important pest, has varied depending on factors such as species, sterilization methods, and numerous other circumstances3,4. In recent years, there has been renewed interest in the SIT for mosquitoes, particularly for Aedes species, due to increased arbovirus outbreaks and the rapid spread of Aedes mosquitoes into new territories5,6,7. While the SIT programs and technical components are still under development for mosquitoes8,9,10,11, and are still relatively small compared to programs for plant pests, significant progress has been made more recently as reviewed in Vreysen et al.12. This progress is crucial for the integrated management of Aedes-borne viral diseases13,14.
The implementation potential of the SIT for mosquito management depends mainly on the capacity to produce sterile males. This requires efficient mass-rearing facilities, with cost-effective methods for insect production, as well as a reliable and reproducible sterilization method with minimal negative effects on male mating competitiveness and other biological quality parameters. Several components are needed to support the testing and implementation of the SIT including baseline data collection to provide information on the biological and ecological parameters of the species, methods for mass rearing, sex separation, sterilization, packing, transport, release and quality control8,9,10,15,16,17,18. Continuous research is also needed for the optimization and standardisation of each step of the technique from small-scale field trials to the operational phase of the SIT programs.
In the SIT, the sterilization step is critical and has been described in numerous studies since 19603. Technical parameters, methods of the sterilization and biological features of Aedes species can significantly affect the sterility of males. Exposure to ionizing radiation (gamma Co-60 or Cs-137, X-ray) is currently the method of choice for rendering insects reproductively sterile19. It has been shown that X-ray provides a practical and effective alternative to gamma-ray for sterilization of insects, are often less expensive, and are subjected to less stringent regulations20,21,22. Ionizing radiation induces dominant lethal mutations in the chromosomes of germ cells of the insect19, significantly affecting the fertility of males22,23,24,25. The higher the absorbed dose, the higher the sterility, and the higher the risk of off-target somatic damage occurring, which can negatively impact insect quality26. Thus, radiation dose presents a trade-off between effective sterilization and the quality of the sterile males27. Mating competitiveness, survival and flight ability of sterile males need to be as close as possible to the wild population28. The optimum dose to ensure sufficient sterility without excessive loss in quality depends on each strain, and each facility-specific set-up. For example, in some facilities, the optimum dose for Aedes albopictus is reported to be between 35 and 40 Gy whereas it is 50 Gy for Aedes aegypti20,24,29,30. Other facilities require 55 Gy and 70 Gy for the two species respectively21,31,32. Dose–response is also affected significantly by atmospheric conditions during irradiation, and particularly oxygen levels have been shown to have significant impact on irradiation outcome25,33. For Ae. albopictus and Ae. aegypti, studies have shown a significant radioprotective effect of oxygen depletion during irradiation procedures25. Biological characteristics of Aedes spp. can also impact radiosensitivity, such as insect life stage and pupal age during irradiation. The maturation of insect reproductive organs and also germ cell development changes with life stage and age, affecting sensitivity to radiation induced somatic damage26. A strong negative correlation has been shown between pupal age and radiosensitivity for Ae. aegypti25 and Ae. albopictus34. For the SIT, it is therefore important to irradiate with a dose sufficient to sterilize the oldest pupae to ensure that no sub-sterile males remain after irradiation33. Other factors such as the nutritional state of pupae, effects of pre-conditioning stress factors, and diapause may additionally influence radioresistance in mosquitoes and have yet to be assessed for Aedes spp19,20,21,22.
Studies on the baseline data collection of pupae sterilization have, for the most part, been performed on small samples, i.e. consisting of a few hundred individuals, and sometimes less. In order to upscale the SIT for field trials on La Reunion Island, the production of around 300 000 sterile males per week is needed6. Thus, the capacity to irradiate a larger number of pupae in bulk is an essential parameter to assess. To date, no method for large scale bulk-irradiation of pupae has been described. Currently, there are few countries working on mass pupae sterilization (China, Italy, Singapore, USA), but there is no detailed information available on the methodologies used in these programs. Effective and standardized mass irradiation methods are thus needed.
As part of the up-scaling process towards a SIT pilot trial against Ae. albopictus on La Reunion6, the aim of the present study was to develop an efficient upscaled irradiation protocol for a weekly production of 300 000 sterile males of Ae. albopictus with the specificities of the only available irradiator on the island. Using available resources of La Reunion, and according to previous studies to determine optimum factors of sterilization for the species, we assessed the effects of exposure environment, pupae density, quantity of water and irradiation dose on the dose–response of Ae. albopictus male pupae. We also evaluated the effect of sample location within the irradiation canister to ensure a consistent and reproducible sterilization method. The long-term objective of this work, which occurred in parallel with the field pilot testing of the SIT on La Reunion, was to acquire sufficient information to develop protocols for the effective and reliable upscaled irradiation of mosquito pupae.
Mean induced sterility spanned from 0.977 in batches of 500 pupae to 0.931 with 4000 pupae following exposure in water, whereas mean induced sterility spanned from 0.959 in batches of 500 pupae to 0.853 with 4000 pupae following exposure in air (Fig. 1). The best model included all fixed effects and the interaction between exposure environment and pupae density. There was a significant effect of the exposure environment (P = 0.0028, Table 1), where irradiation in water showed higher induced sterility than irradiation in air. There was no difference between the induced sterility of 500 pupae and 2000 pupae either in water (P = 0.3155) or in air (P = 0.1005). For larger pupae densities (3000 and 4000), increased pupae density reduced induced sterility (Table 1) and we also observed more variability in induced sterility, especially for irradiation in air. Finally, a significant interaction between pupae density and exposure environment was only observed at a density of 3000, where water led to an increased induced sterility than air (P < 10–3).
Induced sterility of Ae. albopictus irradiated in water or in air increasing pupae densities. The box plot shows the median and upper and lower quartiles.
The best model included all fixed effects without the interaction between irradiation dose and water quantity. The mean induced sterility was higher following irradiation at 40 Gy (P = 0.0000, Table 2) and showed less variability, as compared to irradiation at 35 Gy. There was no effect of the quantity of water, however the mean induced sterility (and upper and lower quartile) was higher with more water (Fig. 2).
Induced sterility of Ae. albopictus following irradiation at 35 Gy or at 40 Gy depending on quantity of water during the irradiation of 2000 pupae in water. The box plot shows the median and upper and lower quartiles.
Mean induced sterility of Ae. albopictus ranged from 0.994 for pupae density of 500 to 0.987 for pupae density of 2500 (Supplementary Fig. S1). There was no difference in the induced sterility between pupae irradiated at a density of 500 and 2000 (P = 0.9399) but increasing the density to 2500 reduced the induced sterility (P = 0.0496). When all extreme data belonging to one particular repetition were removed from the analysis, the difference was even more significant (P = 0.0021).
Mean induced sterility ranged between 0.991 and 0.996 in samples placed throughout the canister and showed more variability in the central locations for a fixed dose of 40 Gy in 130 ml of water (Fig. 3). The location of pupae in the canister did not affect the induced sterility of Ae. albopictus, except for the seventh location, at the top of the canister (P = 0.0083), when location 4 was taken as a reference in the analysis. Central positions in the canister between 3 and 6 showed more variability in induced sterility in adults. There was no difference between locations 1 and 7. However, when removing extreme data from the analysis (all belonging to the same repetition as in experiment 3), no difference was observed for all positions in the canister (P = 0.526).
Induced sterility of Ae. albopictus following the exposure at various locations in the canister for the irradiation of 2000 pupae in 130ml of water at 40 Gy in a full canister. The box plot shows the median and upper and lower quartiles.
The series of experiments reported here have shown that there are different factors that affect induced sterility in Ae. albopictus, which must be taken into consideration when developing a routine irradiation protocol for pupae during an SIT control program.
Exposure in water during pupae irradiation led to higher induced sterility than exposure in air in our experiment. Access to oxygen could explain this result. Generally, mosquito pupae seem to be bimodal breathers, obtaining oxygen via their respiratory trumpets directly from the air when they float on the water surface35,36, and via diffusion of dissolved oxygen (DO) from the water through their cuticle when submerged37,38. Studies have shown depletion of DO in water by Ae. albopictus pupae. When they are enclosed in water with no air bubble at a density of 100 pupae/ml, the pupae depleted the water of DO reaching 50% within 5–6 min and 0.1% DO within 20–25 min33. When pupae are surrounded by an oxygen-poor environment, a protective effect against irradiation effects was demonstrated (water with < 0.5% DO), showing a lower induced sterility in pupae25,33, and similar effects were observed for the irradiation of adults in nitrogen39. In our conditions, the water containing the pupae was renewed just before the treatment and the petri dish offered a large surface area, which allows all pupae to float in a monolayer (except for the density of 4000 pupae) and breathe the ambient air above the water suggesting that pupae had enough oxygen not to incur protection against irradiation. On another hand, when pupae were treated in air, respiration could have been impaired by the fact that pupae were compressed together while lying on the side, especially in treatments with a large number of pupae that could avoid respiration with the trumpets. Moreover, the pupae were not totally dry and were covered in a film of water that could still create small pockets of hypoxia. A low density of pupae per ml may optimize the access to the oxygen for pupae, both in water via cuticular respiration, and in air, via access to the surface, minimizing the protective effect of hypoxia. Further experiments are needed to evaluate differences between conditions in air and conditions in water during pupae irradiation on resulting induced sterility and also the effects of handling pupae in bulk for dry conditions in the context of large-scale irradiation.
The number of pupae that can be irradiated in one irradiation event depends on the irradiator’s specificities, and self-contained units are limited by the chamber, or canister size. Each facility requires the optimization of methods depending on the irradiator available. To our knowledge, no irradiation study had been previously performed on the effects of pupae density during irradiation. We demonstrated a decrease of induced sterility with the increase in pupal density, when pupae density exceeded 3000/petri dish. The larger the sample size and volume of material, the more heterogeneous the absorbed dose. A biological hypothesis could be that with higher densities of pupae, more O2 is depleted in the surrounding water, providing some radioprotection in the pupae. These two hypotheses could be investigated respectively be studying effect of different location in the petri dishes and effect of quantity of water (with measuring the dissolved oxygen) on the induced sterility. In our experiment, no effect of the density on the induced sterility was observed for irradiation up to 2000 pupae, which clearly appeared to be the best pupae density for pupae irradiation at 35 Gy.
In our experiment, we showed that irradiation of pupae in a larger quantity of water provided no significant effect on induced sterility in the adults, at both 35 Gy or at 40 Gy. However, at 40 Gy, the mean induced sterility (and upper and lower quartile) with 130 ml of water was higher than with 30 ml of water. The absence of significant effect of water quantity on pupal irradiation allows us to hypothesize that the amount of oxygen present in 30 ml of water is sufficient to irradiate 2000 pupae for a duration of 5,33 or 6,05 min to achieve 35 Gy and 40 Gy respectively. Further experiments with the measurement of oxygen depletion would allow us to test this hypothesis. However, in the context of a facility, and with the parameters of the irradiator tested on La Reunion, we recommend using the largest quantity of water, i.e. 130 ml, with the assumption that this provides a larger quantity of available oxygen to prevent total oxygen depletion, even if there is a delay in the irradiation procedure. Future experiments will test this hypothesis. Furthermore, the use of a large petri dish does not add complexity to the handling of the pupae during irradiation and doesn't increase handling time.
The optimal irradiation dose for Aedes albopictus pupae has been reported to be between 35 and 42 Gy in water20,24,29,30. It is important that insects used for the SIT are not over-dosed, as this can lead to a decrease in male biological quality27. We thus started with the lowest doses of 35, and 40 Gy to assess whether we could achieve the target sterility in the mosquito strain, and irradiation set-up used. We found that 40 Gy was more reliable in reaching the target sterility (above 99%). Previous studies have shown that survival of male pupae and flight ability in adults were not affected by increasing the dose up to 50 Gy20,24. In our experiment and with our irradiator, 40 Gy clearly appeared to be the best irradiation dose for upscaled irradiation of pupae, per batch of 2000 pupae in water.
Previous experiments have highlighted factors that affect dose response in pupae sterilised at small scale. In the context of optimizing the production of sterile males in a factory, we wanted to maximize pupae density, presenting a new factor. We found a difference between the irradiation of 2000 and 2500 pupae in 130 ml of water at 40 Gy. Thus, it was not possible to increase the density of irradiated pupae without effects on the irradiation outcome. A decrease in radiation effects as pupal densities increase may be correlated to the decrease in DO as more pupae respire in the available volume of water. With the Reunion irradiator specificities, we could thus irradiate only 14 000 pupae per irradiation cycle, a result that was used to plan and implement field releases of sterile males within our control program.
By testing the induced sterility of males irradiated at various locations in the canister/petri dishes, we found that overall, there was a good dose uniformity, and only position 7 had a slightly higher dose (as seen by higher sterility), which corresponds to the dose map performed during irradiator maintenance (Supplementary Fig. S2). Quality assurance is an important part of any successful SIT program for area-wide integrated pest management19.
Standardizing the sterilization process for mosquito pupae remains a challenge, and the irradiation at adult stage is becoming the preferred method. Irradiation protocols for adults are under development and require immobilization prior to compacting and packing the samples, either by chilling or the use of anaesthetics such as nitrogen39,40. However, the sterilization method defined by highlighted factors in previous experiments showed consistent and reproducible results that can be used to irradiate pupae, thus offering an alternative to countries considering pupae irradiation. With these settings, the target of 300,000 sterile males per week could be fulfilled with a total irradiation time of about 2h20 per week. If such an irradiator was fully available to the SIT program, the upscaled irradiation protocol presented here may allow producing more than 5 million sterile males per week considering 5 working days of 8 h.
The Aedes albopictus strain used for the experiment originated from field egg collections in the northern region of Reunion Island at Saint-Marie in 2014. The strain has been maintained under laboratory conditions at the CYROI, Saint-Denis, Reunion Island, in a climate-controlled insectary at 27 ± 2 °C, 75 ± 2% relative humidity (RH), photoperiod of 12: 12 h (light: dark).
Adults were kept in standard rearing cages (30 × 30 × 30 cm, Bugdorm, Taiwan) composed of 1125 females and 375 males with continuous access to 5% (wt:vol) sucrose solution. After having been deprived of sugar for 5 h, females were offered blood meals with defibrinated fresh bovine blood for 30 min using the Hemotek® membrane feeding system (plate of 10 cm diameter and 20 ml total blood capacity). Egg collections were performed two days after blood meals. Females oviposited in plastic beakers lined with crepe paper (Sartorius Stedim Biotech GmbH, Göttingen, Germany) containing deionized water and placed in the cage. Oviposition cups were removed and filter papers were left to dry for one week in plastic containers covered with an opaque plastic plate at the ambient conditions of the insectary.
Egg papers from one to three months old were placed in 250 ml plastic jars with 225 ml of water for 30 min. 25 ml of hatching solution (0,3% wt:vol acid ascorbic solution) was added to the jars which were then closed for 4 h to allow the optimal decrease of oxygen for hatching. A larvae counter was used to quantify hatched larvae into batches of 4000 larvae, which were then transferred to plastic trays (30 by 60 by 10 cm) containing 2 L of water. The trays were covered and larvae were fed daily for 4 days with a total of 100 ml of development solution15. Pupae were collected daily, sexed on pupal size dimorphism using a glass pupal sorter and transferred into plastic beakers inside a fresh adult cage for emergence.
All male pupae used in the following experiment were collected until 30 h before irradiation, which means pupae were 30–44 h old. Pupae were sexed based on pupal size dimorphism using different sizes of sieves in water and sex was verified under a stereomicroscope. Females were placed in individual tubes for emergence to ensure sex and virginity for later mating. Cups of male pupae were transported to the irradiation facility and placed in petri dishes just before irradiation. For tests with water, renewed and temperate tap water was used in petri dishes. Several repetitions were performed according to the experiments as described below.
The X-ray irradiator (Actemium Cegelec, BloodXrad 13–69, Le-Plessis-Pâté, France) of the CHU Félix Guyon (Saint-Denis, La Réunion) was used for this experiment. It is the only irradiator on the island. It was programmed to deliver a dose of 35 Gy or 40 Gy for each exposure, with a dose rate of 0.11 Gy/second. A plastic cylindrical box (14 cm high with a diameter of 14.5 cm) served as the irradiation canister, corresponding to 2312 cm2. Petri dishes were stacked inside the canister to increase available surface area for pupae The irradiation chamber and canister are positioned between 2 X-ray tubes (above and below). The irradiator dose rate is verified every year at 15 positions in the canister using an ionization chamber (TM31010) coupled with a UNIDOS electrometer (UNIDOS PTW T10021 n° 000778, Freiburg, Germany) (Supplementary Fig. S2).
The effect of exposure environment and pupae density during irradiation has been assessed and reported for two exposure environments (water and air) and for five pupae densities (500, 2000, 3000 and 4000). Male pupae were placed in a petri dish (8.5 cm × 1.2 cm). For the water environment, 30 ml of tap water was added with 500, 2000, 3000 and 4000 pupae, corresponding to pupae densities of 16.7, 66.7, 100 and 133.3 pupae /ml respectively. For the air environment, a hole (5.8 ± 0.1 cm) was made in the center of the petri dish, covered with a net to allow water to escape. Male pupae were placed in the petri dishes and excess water was removed by placing the petri dishes on an absorbent paper (but not dried). Thus, the pupae were in the air, but still in damp conditions. For irradiation, 5 to 8 stacked petri dishes were placed in the center of the canister and pupae were irradiated with 35 Gy.
The effect of dose and water quantity during the irradiation of 2000 pupae has been assessed and reported for two doses (35 Gy and 40 Gy) and for two quantities of water (30 ml and 130 ml). Classic petri dishes (8.5 cm × 1.2 cm) were used for 30 ml, corresponding to 66.7 pupae/ml; and large petri dishes were used for 130 ml (13.7 cm × 1.7 cm), corresponding to 15.4 pupae/ml. In both petri dish types, water surface was sufficient to allow all of the pupae to remain in a monolayer and to have access to air with sufficient space around them. For each petri dish, pupae remained in a single layer on the surface of the water. For irradiation, 5 to 8 classic petri dishes, or 4 to 7 large petri dishes were stacked and placed at the centre of the canister.
According to the results of experiments 1 and 2, we determined the most suitable exposure environment, pupae density, dose and water quantity for upscaling the irradiation procedure of male pupae of Ae. albopictus. In the context of optimizing the production of sterile males in a factory, we wanted to test whether the use of these two factors (dose and water quantity) could optimize the number of pupae irradiated per petri dish, i.e., the irradiation of more than 2000 but not exceeding 3,000 pupae. The effect of pupae density during irradiation in water at 40 Gy and in large petri dishes (corresponding to 130 ml of water, or 15.4 pupae/ml) has been assessed and reported for 2 pupal densities (500 and 2000) and an intermediate pupal density (2500). For irradiation, 5 to 7 large petri dishes were stacked and placed at the center of the canister and pupae were irradiated with 40 Gy.
The effect of the location of pupae during upscaled irradiation has been assessed and reported for 7 positions within the petri dishes, corresponding to the location of the petri dishes in the canister, from position 1 at the bottom of the canister to position 7 at the top of the canister. In this experiment, the canister was fully loaded. For irradiation, 7 large petri dishes were stacked and set in the canister and pupae were irradiated with 40 Gy in 130 ml of water.
Combined effects of all factors assessed in the previous 4 experiments were determined based on the induced sterility of adults. For each experiment and repetition, a control was provided with non-irradiated male pupae. For each test and control, 150 male pupae were randomly selected and transferred to plastic cups inside a fresh adult cage for emergence with a continuous access to 5% (wt:vol) sucrose solution. 150 virgin females were added to the cage. After 9 days, a fresh blood meal was offered to females over two consecutive days and oviposition cups were placed in the cages four days after blood feeding (see adult rearing section). Oviposition cups were removed after 32 h and the filter papers were left to dry for one week in plastic containers covered with an opaque plastic plate at ambient conditions of the insectary. Eggs were hatched after 17 days (see hatching section). The number of eggs that hatched out of the total number of eggs produced (fertility) was determined over a single gonotrophic cycle under a stereomicroscope. On each paper, 3 bands of a minimum of 350 eggs were counted, at the left, the center and the right. Preliminary analyses have shown non-significant differences between bands for all tests or controls (Generalized linear mixed model fit by maximum likelihood (Laplace Approximation), P > 0.7950). In the study, experimental methods changed between experiments as new information regarding certain parameters became available, leading to some variations in sample size and number of repetitions between the different experiments.
Induced sterility (IS) was calculated for each treatment group as follows: IS = (HRc − HRt)/HRc; where HRt is the hatch rate of the treatment (t) group, and HRc is the hatch rate of the control (c) group. The overall natural (control) fertility of the strain used was 0.92 (± 0.03) in 96 reps, but controls between experiments showed differences, thus we chose to use ponderation to analyse data. Each test IS had been divided by its associated control IS. Statistical analyses were performed using Microsoft Excel (v.16.0, Microsoft, Redmont, WA, USA) and R v4.2.341.
The effect of exposure environment and pupae density during upscaled irradiation on induced sterility was analyzed using a gaussian linear mixed-effects model fit by maximum likelihood, with exposure environment, pupae density and their first order interaction used as fixed effect and repetition as a random effect. The same analysis was used to test the effect of irradiation dose and water quantity during upscaled irradiation on induced sterility, but without a significant interaction between the two fixed effects.
The effect of the optimization of pupae density during irradiation on induced sterility was analysed using a gaussian linear mixed-effects model fit by maximum likelihood, with location as a fixed effect and repetition as a random effect. The same analysis was used to test the effect of location in the entire canister during irradiation on induced sterility.
The best model in all analyses was selected based on the lowest corrected Akaike information criterion (AICc), and the significance of fixed effects was tested using the likelihood ratio test42,43. All significant differences are based on P < 0.05.
No specific permit was required for the study reported herein. The cow blood used for mosquito feeding was handled in strict accordance with the health regulations and guidelines of the local Animal and Environmental Health Protection protocol (Ref. ANI.ENV.ENR 002) and in compliance with the authorization issued by a Prefectural decree No. SALIMPSPAE -2021-214-D for the use of animal by-products for research purposes.
The data generated and analyzed during the current study are available from the corresponding authors on reasonable request.
Knipling, E. F. Possibilities of insect control or eradication through the use of sexually sterile males. J. Econ. Entomol. 48, 459–462 (1955).
Dyck, V. A., Hendrichs, J. & Robinson, A. S. Sterile Insect Technique: Principles and Practice in Area-Wide Integrated Pest Management (Springer, 2006).
Dame, D. A., Curtis, C. F., Benedict, M. Q., Robinson, A. S. & Knols, B. G. J. Historical applications of induced sterilisation in field populations of mosquitoes. Malar. J. 8, S2 (2009).
Article PubMed PubMed Central Google Scholar
Klassen, W. & Curtis, C. F. History of the sterile insect technique. In Sterile Insect Technique: Principles and Practice in Area-Wide Integrated Pest Management (eds Dyck, V. A. et al.) 3–36 (Springer Netherlands, 2005).
Martín-Park, A. et al. Pilot trial using mass field-releases of sterile males produced with the incompatible and sterile insect techniques as part of integrated Aedes aegypti control in Mexico. PLoS Negl. Trop. Dis. 16, e0010324 (2022).
Article PubMed PubMed Central Google Scholar
Gouagna, L.-C. et al. Strategic approach, advances, and challenges in the development and application of the SIT for area-wide control of Aedes albopictus mosquitoes in Reunion Island. Insects 11, 770 (2020).
Article PubMed PubMed Central Google Scholar
Becker, N. et al. Integrated control of Aedes albopictus in Southwest Germany supported by the sterile insect technique. Parasit. Vectors 15, 1–19 (2022).
Mastronikolos, G. D. et al. Quality control methods for Aedes albopictus sterile male transportation. Insects 13, 179 (2022).
Article PubMed PubMed Central Google Scholar
Kavran, M. et al. Optimization of Aedes albopictus (Diptera: Culicidae) mass rearing through cost-effective larval feeding. Insects 13, 504 (2022).
Article PubMed PubMed Central Google Scholar
Gómez, M. et al. exploring conditions for handling packing and shipping Aedes aegypti males to support an SIT field project in Brazil. Insects 13, 871 (2022).
Article PubMed PubMed Central Google Scholar
Dobson, K. L. et al. Satellite rearing of Aedes mosquito eggs: Synchronized empirical test of a novel mass rearing model. J. Am. Mosq. Control Assoc. 39, 12–17 (2023).
Vreysen, M. J. et al. The insect pest control laboratory of the Joint FAO/IAEA programme: Ten years (2010–2020) of research and development, achievements and challenges in support of the sterile insect technique. Insects 12, 346 (2021).
Article PubMed PubMed Central Google Scholar
Roiz, D. et al. Integrated Aedes management for the control of Aedes-borne diseases. PLoS Negl. Trop. Dis. 12, e0006845 (2018).
Article PubMed PubMed Central Google Scholar
World Health Organization & UNICEF. Global vector control response 2017–2030 (2017).
Damiens, D. et al. Aedes albopictus adult medium mass rearing for sit program development. Insects 10, 246 (2019).
Article PubMed PubMed Central Google Scholar
Gunathilaka, P. et al. Determination of the efficiency of diets for larval development in mass rearing Aedes aegypti (Diptera: Culicidae). Bull. Entomol. Res. 108, 583–592 (2018).
Article CAS PubMed Google Scholar
Sánchez-Aldana-Sánchez, G. A. et al. Release of sterile Aedes aegypti mosquitoes: chilling effect on mass-reared males survival and escape ability and on irradiated males sexual competitiveness. Sci. Rep. 13, 3797 (2023).
Article ADS PubMed PubMed Central Google Scholar
Yamada, H. et al. Radiation dose-rate is a neglected critical parameter in dose–response of insects. Sci. Rep. 12, 6242 (2022).
Article ADS CAS PubMed PubMed Central Google Scholar
Bakri, A., Mehta, K. & Lance, D. R. Sterilizing insects with ionizing radiation. In Sterile Insect Technique: Principles and Practice in Area-Wide Integrated Pest Management (eds Dyck, V. A. et al.) 233–268 (Springer Netherlands, 2005). https://doi.org/10.1007/1-4020-4051-2_9.
Yamada, H., Parker, A. G., Oliva, C. F., Balestrino, F. & Gilles, J. R. L. X-ray-induced sterility in Aedes albopictus and male longevity following irradiation. J. Med. Entomol. 51, 811–816 (2014).
Article CAS PubMed Google Scholar
Yamada, H. et al. Suitability of Raycell MK2 blood X-ray irradiator for the use in the sterile insect technique: dose response in fruit flies, tsetse flies and mosquitoes. Insects 14, 92 (2023).
Article PubMed PubMed Central Google Scholar
Chen, C. et al. Developing the radiation-based sterile insect technique (SIT) for controlling Aedes aegypti: Identification of a sterilizing dose. Pest Manag. Sci. 79, 1175–1183 (2023).
Article CAS PubMed Google Scholar
FAO/IAEA. Guidelines for small scale Irradiation of mosquito pupae in SIT programs. 1.0 http://www-naweb.iaea.org/nafa/ipc/public/2020-Guidelines-for-Irradiation.pdf (2019).
Bond, J. G. et al. Optimization of irradiation dose to Aedes aegypti and Ae albopictus in a sterile insect technique program. PloS One 14, e0212520 (2019).
Article CAS PubMed PubMed Central Google Scholar
Yamada, H. et al. Identification of critical factors that significantly affect the dose-response in mosquitoes irradiated as pupae. Parasit. Vectors 12, 1–13 (2019).
Lance, D. R. & McInnis, D. O. Biological basis of the sterile insect technique. In Sterile Insect Technique: Principles and Practice in Area-Wide Integrated Pest Management (eds Dyck, V. A. et al.) 69–94 (Springer Netherlands, 2005). https://doi.org/10.1007/1-4020-4051-2_3.
Calkins, C. O. & Parker, A. G. Sterile insect quality. In The Sterile Insect Technique: Principles and Practice in Area-Wide Integrated Pest Management (eds Dyck, V. A. et al.) 269–296 (Springer, 2005).
Bouyer, J. & Vreysen, M. J. Yes, irradiated sterile male mosquitoes can be sexually competitive!. Trends Parasitol. 36(11), 877–880 (2020).
Article CAS PubMed Google Scholar
Du, W. et al. Comparison between pupal and adult X-ray radiation, designed for the sterile insect technique for Aedes albopictus control. Acta Trop. 199, 105110 (2019).
Qiu, J. et al. A preliminary experimental study of X-ray-induced sterility in Aedes albopictus pupae. Zhongguo Meijie Shengwuxue Ji Kongzhi Zazhi Chin J. Vector Biol. Control 30, 422–426 (2019).
Somda, N. S. B. et al. Response of male adult Aedes mosquitoes to gamma radiation in different nitrogen environments. Front. Bioeng. Biotechnol. https://doi.org/10.3389/fbioe.2022.942654 (2022).
Yamada, H. et al. Radiation dose-fractionation in adult Aedes aegypti mosquitoes. Parasite 30, 5 (2023).
Article PubMed PubMed Central Google Scholar
Yamada, H. et al. The role of oxygen depletion and subsequent radioprotective effects during irradiation of mosquito pupae in water. Parasit. Vectors 13, 1–10 (2020).
Balestrino, F. et al. γ ray dosimetry and mating capacity studies in the laboratory on Aedes albopictus males. J. Med. Entomol. 47, 581–591 (2014).
Ha, Y.-R., Yeom, E., Ryu, J. & Lee, S.-J. Three-dimensional structures of the tracheal systems of Anopheles sinensis and Aedes togoi pupae. Sci. Rep. 7, 44490 (2017).
Article ADS PubMed PubMed Central Google Scholar
Nelson, M. J. Aedes aegypti: biology and ecology. (1986).
Clements, A. N. The Biology of Mosquitoes, Volume 1. Development, Nutrition and Reproduction Vol. 1 (Chapmann & Hall, 1992).
Wigglesworth, V. The function of the anal gills of the mosquito larva. J. Exp. Biol. 10, 16–26 (1933).
Yamada, H. et al. Effects of chilling and anoxia on the irradiation dose-response in adult aedes mosquitoes. Front. Bioeng. Biotechnol. https://doi.org/10.3389/fbioe.2022.856780 (2022).
Article PubMed PubMed Central Google Scholar
Balestrino, F. et al. Mass irradiation of adult Aedes mosquitoes using a coolable 3D printed canister. Sci. Rep. 14, 4358 (2024).
Article CAS PubMed PubMed Central Google Scholar
R Core Team. R: A Language and Environment for Statistical Computing. (R Foundation for Statistical Computing, 2021).
Hurvich, C. M. & Tsai, C.-L. Model selection for extended quasi-likelihood models in small samples. Biometrics 51, 1077–1084 (1995).
Article CAS PubMed Google Scholar
Burnham, K. P. & Anderson, D. R. Model Selection and Multimodel Inference: A Practical Information-Theoretic Approach 2nd edn. (Springer-Verlag, 2002).
We are thankful to the the EFS of the Félix Guyon Hospital Center in St-Denis for providing the necessary access to the irradiation facility and for their input to various aspects of this study. We thank the SICABAT slaughterhouse in Saint-Pierre for providing fresh bovine blood used for female mosquitoes feeding. The assistance received from the IAEA’s IPCL in the form of advice for the establishment of the mosquito colony and the mass production system is deeply acknowledged. We are grateful to all the students and technical staff for all the effort and their significant contributions to all research activities within the SIT program since its start in 2009.
This research was funded by the ERDF program, grant numbers “ERDF No. 2012-32122” and “GURDTI 2017-0583-0001899” within the 2007–2013 and 2014–2020 frameworks—Action 1.05 “Strengthening the Health and biotechnology Innovation”, and The French Ministry of Health and the Ministry of Overseas Territories.
UMR Mivegec (Infectious Diseases and Vectors: Ecology, Genetics, Evolution and Control), IRD-CNRS-Univ.Montpellier, IRD Reunion Representation – PTU, 97495, Sainte Clotilde Cedex, Réunion, France
Lucie Marquereau, David Damiens, Antonin Leclercq, Brice Derepas, Cécile Brengues, Brice William Dain, Quentin Lejarre, Mickael Proudhon & Louis Clément Gouagna
Insect Pest Control Laboratory, Joint FAO/IAEA Programme of Nuclear Techniques in Food and Agriculture, IAEA Vienna, Wagramer Strasse 5, 1400, Vienna, Austria
Hanano Yamada & Jeremy Bouyer
ASTRE, CIRAD, INRAE, University of Montpellier, 34398, Montpellier, France
ASTRE, CIRAD, INRAE, University of Montpellier, CYROI Technological Platform, Sainte-Clotilde, Réunion, France
UMR Mivegec, IRD-Occitanie Regional Delegation, 34394, Montpellier, France
You can also search for this author in PubMed Google Scholar
You can also search for this author in PubMed Google Scholar
You can also search for this author in PubMed Google Scholar
You can also search for this author in PubMed Google Scholar
You can also search for this author in PubMed Google Scholar
You can also search for this author in PubMed Google Scholar
You can also search for this author in PubMed Google Scholar
You can also search for this author in PubMed Google Scholar
You can also search for this author in PubMed Google Scholar
You can also search for this author in PubMed Google Scholar
You can also search for this author in PubMed Google Scholar
D.D., L.M. conceptualized the experimental designs for the experiments. L.M., D.D., A.L., B.De. carried out the experiments. C.B., B.Da., Q.L., M.P. participated in the lab operations. JB and LM carried out the statistical analyses. L.M. drafted the original manuscript. H.Y. contributed significantly to the written content of the manuscript. L.C.G provided project administration and coordination, supervised the project and acquired funding. All authors read and approved the final manuscript.
Correspondence to Lucie Marquereau or Louis Clément Gouagna.
The authors declare no competing interests.
Springer Nature remains neutral with regard to jurisdictional claims in published maps and institutional affiliations.
Open Access This article is licensed under a Creative Commons Attribution 4.0 International License, which permits use, sharing, adaptation, distribution and reproduction in any medium or format, as long as you give appropriate credit to the original author(s) and the source, provide a link to the Creative Commons licence, and indicate if changes were made. The images or other third party material in this article are included in the article's Creative Commons licence, unless indicated otherwise in a credit line to the material. If material is not included in the article's Creative Commons licence and your intended use is not permitted by statutory regulation or exceeds the permitted use, you will need to obtain permission directly from the copyright holder. To view a copy of this licence, visit http://creativecommons.org/licenses/by/4.0/.
Marquereau, L., Yamada, H., Damiens, D. et al. Upscaling irradiation protocols of Aedes albopictus pupae within an SIT program in Reunion Island. Sci Rep 14, 12117 (2024). https://doi.org/10.1038/s41598-024-62642-7
DOI: https://doi.org/10.1038/s41598-024-62642-7
Anyone you share the following link with will be able to read this content:
Sorry, a shareable link is not currently available for this article.
Provided by the Springer Nature SharedIt content-sharing initiative
By submitting a comment you agree to abide by our Terms and Community Guidelines. If you find something abusive or that does not comply with our terms or guidelines please flag it as inappropriate.
Scientific Reports (Sci Rep) ISSN 2045-2322 (online)
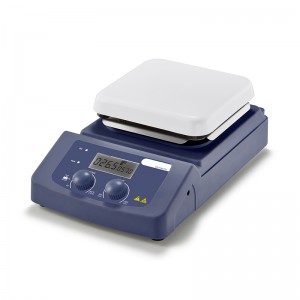
Centrifuge Sign up for the Nature Briefing: Translational Research newsletter — top stories in biotechnology, drug discovery and pharma.